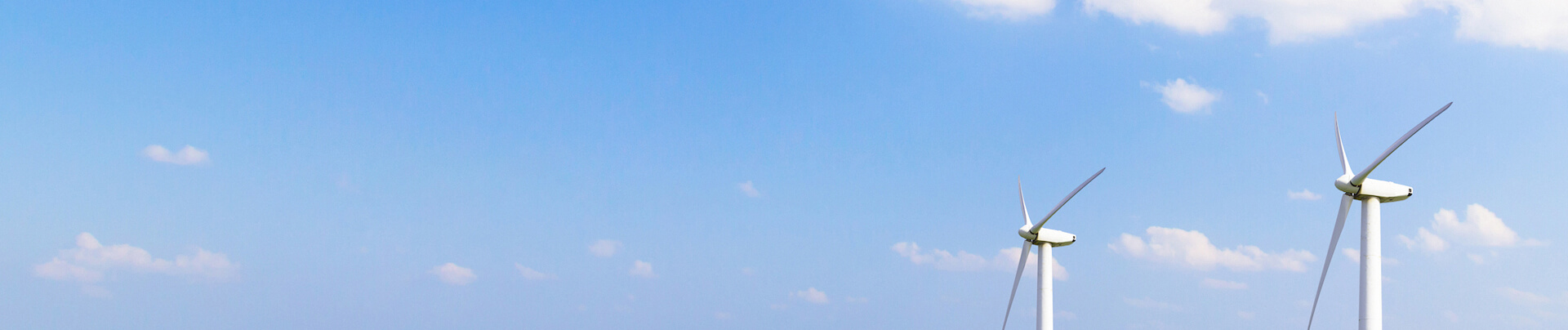
To Understand
Your Project Goals and Outputs
As the power density of electronic devices continues to rise, heat dissipation design has become a key link affecting system reliability and lifespan. As a professional manufacturer with 19 years of experience in thermal management, we combine typical engineering cases with multi-physics field simulation experience to deeply analyze the five major technical misunderstandings that engineers tend to overlook in high-power scenarios, and provide solutions that conform to industry practices.
Misunderstanding 1: Abuse of high thermal conductivity materials, calculation deviation of interface thermal resistance
Typical problem: Excessive pursuit of theoretical values of thermal conductive materials, ignoring the engineering reality of interface contact thermal resistance.
Case analysis: A certain automotive laser radar heat dissipation module uses a pure copper substrate (thermal conductivity 401W/m·K), but the coating thickness of the thermal interface material (TIM) is not accurately controlled (designed 0.1mm, actual fluctuation ±0.05mm), and the measured contact thermal resistance reaches 0.6°C·cm²/W, causing the FPGA junction temperature to exceed the standard by 22%. After switching to preformed thermal pads (tolerance ±0.02mm, thermal resistance <0.03°C·cm²/W) and combining with vacuum sintering process, the junction temperature is reduced by 17°C, and the MTBF (mean time between failures) is increased to 100,000 hours.
Optimization plan:
Material selection: According to the ASTM D5470 standard, the thermal resistance of TIM is measured, and phase change materials or metal-filled elastomers are preferred.
Process control: Use automatic dispensing equipment (accuracy ±3%) to ensure thickness tolerance <10% and avoid air gaps.
Misunderstanding 2: Mismatch of airflow organization in air cooling system, local hot spots out of control
Typical problem: blindly stacking the number of fans, ignoring the flow channel impedance matching and boundary layer separation effect.
Engineering example: A data center GPU cluster uses 4 12038 fans (air volume 200CFM), but due to improper design of the air guide angle (90° right angle bend), the actual effective air volume is only 65% of the nominal value, and the local temperature difference reaches 40°C. After CFD simulation optimization, the air guide is changed to a gradually expanding flow channel (diffusion angle <7°), and a staggered fin layout is adopted (the spacing gradient increases from 2mm to 3.5mm), the overall wind resistance is reduced by 30%, and the hot spot temperature difference is controlled within 8°C.
Core strategy:
Flow field design: Wind tunnel testing is carried out according to ISO 5801 standard to optimize the inlet and outlet area ratio (recommended 1:1.2).
Dynamic control: Based on the Q-H curve of the PWM fan, the system impedance point is matched to the highest efficiency range (usually 60-80% of the maximum air volume).
Misconception 3: Failure of environmental protection design accelerates material aging
Typical problem: Only focusing on initial heat dissipation performance, ignoring the effects of long-term environmental stress such as humidity, heat, dust, and salt spray.
Case analysis: An offshore wind power converter uses a 6061 aluminum alloy heat sink (surface anodized). After running in a salt spray environment for 6 months, the oxide layer peeled off, causing the contact thermal resistance to increase by 50%, and the IGBT failure rate to surge. Switching to 5052 aluminum alloy (salt spray resistance >1000h) and spraying polyurethane three-proof paint (thickness 50μm, in line with IEC 60068-2-11 standard), the 3-year field failure rate dropped by 75%.
Design points:
Coating technology: Plasma spraying Al2O3 ceramic coating (thermal conductivity 30W/m·K, temperature resistance >800°C) is used in high-temperature scenarios.
Sealing structure: IP69K protection level (double-pass silicone seal + pressure relief valve) to ensure zero intrusion of dust/water vapor.
Misconception 4: Lack of transient heat load modeling and improper selection of phase change materials
Typical problem: The heat dissipation solution designed by steady-state simulation cannot cope with millisecond-level power pulses.
Measured data: A solid-state power amplifier is subjected to a 2ms@2000W pulse load in a 5G base station. The transient temperature rise of the traditional aluminum substrate solution reaches 55°C, while the microcapsule phase change material (paraffin/graphene composite, phase change latent heat 220J/g) combined with transient thermal simulation (ANSYS Icepak transient step length 1ms) is used to control the temperature rise to 18°C, meeting the MIL-STD-810G impact test requirements.
Dynamic management technology:
Phase change packaging: The honeycomb structure is used to encapsulate the phase change material (porosity>70%) to avoid liquid leakage and improve heat diffusion efficiency.
Model calibration: The simulation boundary conditions are corrected based on infrared thermal imaging (sampling rate 100Hz), and the error is <±1.5°C
Misconception 5: TCO evaluation is one-sided and ignores hidden operation and maintenance costs
Typical problem: Only the initial hardware cost is compared, and the energy consumption/maintenance/downtime losses are not quantified.
Cost comparison: A semiconductor factory originally used air-cooled cabinets (initial cost of 500,000 yuan), with an annual electricity bill of 1.2 million yuan (PUE=1.8). After upgrading to two-phase immersion liquid cooling (initial cost of 1.8 million yuan), the annual electricity bill dropped to 450,000 yuan (PUE=1.05), and due to the reduction of clean room air conditioning load, the annual comprehensive savings reached 900,000 yuan, and the incremental investment can be recovered in 2.1 years (IRR>25%).
Decision model:
Refined modeling: Introduce Monte Carlo simulation to quantify the impact of equipment failure rate (Weibull distribution) on TCO.
Policy superposition: Combined with carbon tax mechanisms (such as EU CBAM), the carbon emissions of liquid cooling systems over their entire life cycle are reduced by 60%, and an additional subsidy of 15% is obtained
Conclusion: Upgrading the thermal design paradigm from experience-driven to data-driven
Standardized process: Introduce the JESD51-14 thermal test standard in the conceptual design stage to avoid rework later (the project cycle of a certain optical module is shortened by 30%).
Multidisciplinary collaboration: Through COMSOL Multiphysics coupling electromagnetic loss (HFSS extraction) and thermal strain field, the heat dissipation efficiency of a radar TR component is improved by 40%.
Intelligent operation and maintenance: Deploy fiber grating temperature sensors (accuracy ±0.1°C) and digital twin platforms to achieve fault prediction (unexpected downtime of a supercomputing center is reduced by 90%).
Through rigorous engineering thinking and full-link data verification, thermal design engineers can accurately avoid "invisible killers" and lay the foundation for high-reliability systems. As industry practitioners, we are committed to providing closed-loop solutions from materials to systems, and jointly address thermal management challenges with customers.
We will regularly update you on technologies and information related to thermal design and lightweighting, sharing them for your reference. Thank you for your attention to Walmate.
1- Special tolerance requirements for key parts
In the manufacturing of EV battery trays and Liquid Cooling Energy Storage Battery Pack Enclosure, the tolerance control of key connection surfaces and interfaces directly affects the sealing, heat dissipation efficiency and assembly accuracy.
a. Liquid cooling plate installation surface
The liquid cooling plate is the core component of the battery thermal management system. The flatness of its installation surface directly affects the sealing of the coolant flow channel. If the flatness is out of tolerance, it will cause uneven compression of the silicone gasket, causing local leakage or increased thermal resistance.
b. Welding seam misalignment
The battery tray often uses an aluminum alloy welding structure. Welding seam misalignment will cause stress concentration and reduce fatigue life. Especially for scenarios with high requirements for Battery Pack Enclosure airtightness (such as IP67), misalignment must be strictly controlled.
c. Battery module positioning hole
The positioning hole is used to accurately fix the battery module. Excessive tolerance will cause module misalignment, causing electrical connection failure or mechanical vibration risks.
d. Fixing bolt hole position
The bolt hole is used to connect the Battery Pack Enclosure to the chassis. Position deviation will cause assembly stress and even cause bolt breakage.
e. Battery Pack Enclosure edge straightness
The Battery Pack Enclosure edge straightness affects the assembly of the upper and lower covers and the IP protection level, especially for laser-welded enclosures, where the edge needs to be used as a reference for laser head tracking.
2-The impact of dimensional tolerance on manufacturing efficiency
The impact of dimensional tolerance on manufacturing efficiency is mainly reflected in its balance between processing flow, cost control and product quality. Reasonable tolerance design can not only ensure product function, but also optimize production rhythm. The impact of reasonable control of dimensional tolerance on manufacturing efficiency is mainly reflected in the following aspects:
a. Balance between processing accuracy and production cost
· Although strict dimensional tolerance can improve sealing and assembly accuracy, high-precision processing equipment and complex processes are required, which significantly increases equipment investment and processing time.
· Excessive pursuit of accuracy may lead to increased processing costs. Strict tolerances need to be set in key functional parts, and tolerances should be appropriately relaxed in non-critical areas to reduce costs.
b. Control of rework rate and scrap rate
· Reasonable tolerance design can reduce rework caused by dimensional deviation.
· Welding deformation is the main problem in battery tray manufacturing. The use of CMT cold welding process can optimize heat input, reduce deformation after welding, and shorten the rework cycle.
c. Modular and standardized production
· Through standardized components, tolerance requirements are concentrated on local replaceable parts, reducing the overall processing difficulty.
· Standardized tolerance design can also support multi-model compatibility and reduce the need for customized processing.
3-Industry standards and practical applications of flatness control
For new energy vehicle battery trays, industry standards have strict requirements:
a. Flatness standard: The flatness of the liquid cooling plate installation surface must be ≤0.2mm, the flatness of the bottom support plate must be ≤0.5mm/m², and the flatness error of the frame after welding must be ≤0.8mm6.
b. Manufacturing process optimization: CNC precision milling, CMT cold welding and vibration aging treatment (VSR), combined with laser interferometer online detection, reduce the risk of deformation.
c. Material selection: It is recommended to use high-strength aluminum alloys such as 6061, with a yield strength of ≥1180MPa, taking into account both lightweight and structural stability.
4- Tolerance adjustment strategy driven by materials and processes
a. Material innovation drives lightweighting
Using lightweight high-strength aluminum alloys (such as 6061) and composite materials (silicon carbide reinforced aluminum matrix), combined with anodizing and insulating coating, to achieve 30% weight reduction and improve corrosion resistance, adapting to the heat dissipation requirements of liquid cooling systems.
b. Process collaboration improves manufacturing accuracy
One-piece extrusion molding + stir friction welding (FSW) reduces the number of welds, CMT cold welding and laser welding control thermal deformation.
c. Simulation detection closed loop to ensure reliability
CAE simulation optimizes the layout of reinforcement ribs, laser scanning and helium leak detection technology realize full process monitoring, modular design is compatible with ±5mm tolerance, reducing costs and increasing efficiency.
We will regularly update you on technologies and information related to thermal design and lightweighting, sharing them for your reference. Thank you for your attention to Walmate.
1- Battery tray / energy storage pack box aluminum alloy welding process characteristics
In the manufacturing of new energy liquid-cooled Pack boxes, battery trays and other products, liquid cooling channels and multi-cavity structures are typical complex design features (as shown in Figure 1). Such structures often include the following features:
Figure 1: Typical design of a battery tray
Three-dimensional space interlacing: the flow channels are distributed in a serpentine shape, with a large number of spatial turning points;
Multi-level connection: the main cavity and sub-cavity are connected by thin-walled partitions (thickness 2-3mm);
Miniaturization features: small flow channel cross-section size (the minimum wall thickness of the aluminum profile flow channel is 1.2mm) and poor welding accessibility.
2- Difficulty analysis of combining multiple welding technologies in battery tray manufacturing
In the practice of battery tray manufacturing, it is necessary to combine multiple welding technologies, such as the coordinated application of friction stir welding (FSW), cold metal transition welding (CMT), traditional melting welding (TIG/MIG) and other technologies. This combination aims to take into account lightweight, strength, sealing and production efficiency, but it also faces the following core difficulties:
a. The problem of coordinated heat input of multiple processes
Different welding technologies (such as FSW and laser welding) have large differences in heat input, which can easily cause material performance mutations and thermal deformation, and need to be solved by optimizing the welding sequence and parameter adjustment.
b. Equipment compatibility and efficiency bottleneck
Equipment switching is complex and parameter synchronization accuracy requirements are high. Modular workstation design and intelligent welding machines are required to improve collaborative efficiency and reduce cycle time.
c. Challenges of balancing sealing strength and cost
The risk of leakage at the intersection of multiple welds is high (accounting for 70% of defects), and redundant sealing + topology optimization design is required.
3-Engineering practice: Battery tray, energy storage liquid cooling PACK box multi-welding process coordination
a. Weld scar position planning
· Welding position optimization: In the product design stage, the rationality of the welding position should be fully considered to avoid weld scars appearing in key parts or areas that affect product functions. For example, adjust the gap between the front frame and the water nozzle to prevent weld scar interference; optimize the welding position of the water nozzle to reduce the impact on product performance.
· Matching with product structure: The welding position should match the product structure to ensure that the weld can withstand various loads and stresses during the use of the product. For example, in the liquid cooling plate project, the structural stability and reliability of the product are improved by rationally planning the welding position.
· Easy to operate and detect: The welding position should be convenient for operators to perform welding operations and subsequent quality inspections. For example, avoid welding in locations that are difficult to reach or have poor visibility to improve welding efficiency and quality.
b. Weld scar control
· Weld scar size: The size of the weld scar needs to be strictly controlled. Generally, the weld scar height is required to be within the range of 4/6mm, and the weld scar does not exceed the plane to ensure the appearance and assembly accuracy of the product. For example, in the liquid cooling plate project, the size of the weld scar was successfully controlled by adjusting the welding parameters and process, avoiding the impact of the weld scar on product performance and quality.
· Weld scar shape: The weld scar shape should be as regular as possible to avoid excessive melting or incomplete fusion. By optimizing welding parameters and operating techniques, the weld scar shape can be effectively improved and the weld quality can be improved.
· Grinding treatment: For the concave weld marks produced at the friction welding position, grinding treatment is used to ensure a smooth surface to meet the product appearance and performance requirements.
c. Welding sequence optimization
· Partition welding: For large products, the method of partition welding can effectively control the overall deformation. By dividing the product into several areas and welding them in sequence, the heat concentration during the welding process can be reduced and the risk of thermal deformation can be reduced.
· Step-by-step correction: During the welding process, the step-by-step correction method is used to adjust the welding deformation in time. By continuously measuring and correcting the deformation of the product during the welding process, the dimensional accuracy and shape of the final product are ensured to meet the design requirements.
· Optimize the welding path: Rationally plan the welding path to avoid repeated welding in the same area and reduce heat input. For example, in the liquid cooling plate project, the heat input during the welding process was reduced and the welding quality was improved by optimizing the welding path.
d. Deformation collaborative control
The liquid cooling plate undergoes thermal deformation during the welding process, resulting in a decrease in the dimensional accuracy of the product.
Optimization measures:
· Use partition welding and step-by-step correction methods to control the overall deformation.
· Optimize welding parameters, reduce current and voltage, and reduce heat input.
· Use fixtures to fix the product to reduce movement and deformation during welding.
Use symmetrical welding method to make welding heat evenly distributed.
We will regularly update you on technologies and information related to thermal design and lightweighting, sharing them for your reference. Thank you for your attention to Walmate.
CTC (Cell-to-Chassis) technology has attracted much attention because it can significantly improve the range and space efficiency of electric vehicles, but whether it can truly replace traditional battery technology is still controversial. The core bottleneck on the manufacturing side - the battery tray welding process - has become the key to determining the future of this technology: complex structural design, multi-material compatibility, and stringent thermal management requirements have put traditional welding technology to a huge test. Starting from the perspective of front-line engineers, combining industry trends and engineering practices, this article analyzes the potential and limitations of CTC technology, and explores how welding technology has become the "touchstone" of this technological revolution.
1-The "Ideal and Reality" of CTC Technology
CTC technology can theoretically reduce redundant structural parts and improve energy density by integrating the battery cell directly into the chassis, but the feasibility of its large-scale application is facing realistic challenges:
Car companies' radicalism and caution: Tesla, BYD and other leading companies have launched CTC solutions, but more manufacturers are still waiting and watching. Industry data shows that as of February 2025, CTC mass-produced models account for 15-20% of the world, and traditional CTP (Cell-to-Pack) technology is still the mainstream.
The "cost" of improved battery life: Although CTC can increase energy density by 15%-20%, the complexity of the chassis structure leads to an increase in manufacturing costs of 30%-50% (calculated by a consulting agency), and the balance between cost and benefit is not yet clear.
Maintenance economic controversy: The integrated design has caused the battery maintenance cost to soar. Insurance company data show that the replacement rate of battery assemblies after accidents of CTC models is as high as 70%, far higher than the 25% of traditional models.
These contradictions make the future of CTC technology full of variables. Whether the market can break through 20% depends on three major variables: the progress of Tesla Cybertruck mass production; whether the price war among Chinese automakers forces CTC to reduce costs; and the degree of compromise of European regulations on battery repairability. The level of welding technology on the manufacturing side will be the key to determining whether it can cross the gap between laboratory and mass production.
2-Three "life and death barriers" of welding process
For battery tray manufacturers, the implementation of CTC technology needs to overcome three core problems:
a. The game between "micron-level" precision and efficiency
Traditional welding processes can tolerate 0.5mm errors, but CTC trays need to carry more batteries, and the number of seams increases by more than 3 times, and most of them are distributed on curved and irregular parts. Tests by a manufacturer show that when the welding positioning error exceeds 0.15mm, the yield rate of battery cell assembly will drop sharply from 99% to 82%. What's more tricky is that the improvement in precision is often accompanied by a decrease in efficiency-although the accuracy of laser welding is high, the equipment cost is more than 5 times that of traditional welding.
b. The "water and fire" of mixed materials
In order to balance lightweight and strength, CTC trays often use a mixed structure of "aluminum alloy + carbon fiber + special steel". The difference in thermal expansion coefficients of different materials can be up to 20 times (such as aluminum 23μm/m·K vs. carbon fiber 0.8μm/m·K), which is easy to generate internal stress during welding and cause cracking. When a company tried to connect aluminum and carbon fiber, the crack rate of the weld was as high as 18%, far exceeding the industry's acceptable threshold of 3%.
c. "Invisible killer" of the heat-affected zone
High welding temperature may damage the insulating materials or sensor circuits around the battery cell. A car company once increased the self-discharge rate of the battery module by 50% due to improper control of welding heat input. Engineers must control the welding temperature fluctuation within ±15℃ within 0.1 seconds - this is equivalent to installing a "high-precision temperature brake" on the welding gun.
3-The way out: There is no silver bullet, only system innovation
Faced with these challenges, the industry is exploring three breakthrough paths:
a. "Cocktail therapy" of process combination
Laser arc hybrid welding: Combining the accuracy of laser welding with the advantages of arc welding penetration, the welding speed of aluminum alloy is increased by 40%;
Cold metal transition technology (CMT): Through precise heat input control, the area of heat affected zone is reduced by 60%;
Robot intelligent compensation system: Automatically adjust the welding gun path based on real-time weld scanning data, and increase the qualified rate of complex curved surface welding from 75% to 95%.
b. "Pre-compromise" on the material side
Some companies have begun to jointly develop "welding-friendly" composite materials with material suppliers. For example, the welding crack sensitivity of a domestic modified aluminum alloy (aluminum alloy material adjusted by physical, chemical or process means) has been reduced from level 7 to level 3 (according to ISO standards). Although it sacrifices 5% of the lightweight effect, it increases the welding yield to 98%.
4- Engineers’ new proposition: Finding certainty in uncertainty
a. The controversy over CTC technology is essentially a game between “system optimization” and “local limits”:
If the breakthrough speed of welding process is slower than the cost reduction expectations of car companies, CTC may become a niche technology;
If materials, processes, and testing technologies make breakthroughs in a coordinated manner, it is expected to usher in a new era of electric vehicle structural design.
b. For engineers, it is necessary to reconstruct capabilities from two dimensions:
Cross-domain knowledge integration: understand the sensitive threshold of electrochemical properties to welding heat input;
Agile response capability: A case study of a European supplier shows that the team that can complete the optimization of welding parameters of new alloy materials within a week has a 3-fold increase in the probability of obtaining orders.
CTC technology stands at the crossroads of “disruptive innovation” and “mass production trap”. It may not completely replace existing technology, but it is forcing the welding process to evolve towards higher precision, stronger compatibility, and more intelligent control. In this technology marathon, the real winner may not be the earliest car company, but the engineering team that turns “impossible welds” into “standardized interfaces” on the manufacturing side.
We will regularly update you on technologies and information related to thermal design and lightweighting, sharing them for your reference. Thank you for your attention to Walmate.
As CTP (Cell to Pack) technology completely subverts the traditional battery pack structure, the "role" of the battery tray has shifted from passive load-bearing to active integration. Welding technology has become the core of safety and performance. The requirements of lightweight (wall thickness is only 1.5mm), zero pore sealing, and multi-material (aluminum/copper/carbon fiber) integration have caused traditional welding to fall into the dilemma of deformation and defects. The industry is making breakthroughs through material innovation, intelligent quality inspection and process iteration. This article will analyze the disruptive challenges that CTP poses to welding and explore the technical path to high precision and high reliability.
1-Analysis of the changes in the structural design requirements of battery trays due to CTP technology
CTP (Cell to Pack) technology directly integrates the battery cells into the battery pack by eliminating the module structure in the traditional battery pack. This technological innovation puts forward all-round and multi-dimensional upgrade requirements for the structural design of the battery tray. The following is a specific analysis from the perspectives of materials, performance, process, integration, etc.:
(1) Comprehensive improvement of structural strength and shockproof performance
a. Mechanical bearing requirements after eliminating the module:
After CTP technology eliminates the module structure, the battery tray needs to directly bear the support, fixation and external force buffering functions of the battery cell. The traditional module disperses the mechanical load, while the CTP tray needs to absorb the expansion deformation of the battery cell during charging and discharging as a whole (for example, the expansion force of the square battery cell can reach 10-20kN), while resisting the vibration, extrusion and impact load during vehicle driving.
b. Material and structural optimization direction
· Dominance of high-strength aluminum alloy: Early steel was gradually replaced due to its heavy weight, and 6061-T6 became the mainstream. It has high specific strength and strong corrosion resistance, which can meet the dual requirements of lightweight and high rigidity.
· Composite structure design: For example, the "double-frame ring beam" structure of Leapmotor enhances local impact resistance through longitudinal and transverse beam compartments, while using extrusion technology to optimize material distribution and reduce redundant weight.
· Exploration of magnesium-aluminum alloy and carbon fiber: Magnesium-aluminum alloy is 30% lighter than traditional aluminum materials, and carbon fiber composite materials have both high strength and lightweight characteristics, but are currently only used in high-end models due to cost and process maturity.
(2) Requirements for integrated air tightness and thermal management
a. Sealing performance upgrade
After the module is removed, the internal coolant circulation and gas sealing of the battery pack are completely dependent on the tray, and welding defects (such as pores and cracks) may lead to leakage risks.
Figure 1-Battery tray air tightness test
b. Integration of thermal management functions
CTP trays need to integrate components such as liquid cooling plates and thermal conductive adhesives. For example, structural adhesives are used to fix the battery cells and transfer expansion stress, and polyurethane thermal conductive adhesives (thermal conductivity > 1.5 W/m·K) are used for heat conduction between battery cells and with liquid cooling tubes. The amount of adhesive used in a single PACK is more than 50% higher than that in traditional structures. Flow channels need to be designed inside the tray to optimize cooling efficiency while avoiding damage to the sealing caused by the heat affected zone of welding.
(3) Lightweighting and material innovation
a. Material selection trends
Aluminum alloy extrusion and die-casting process: extruded aluminum profiles are used for frame structures (such as Tesla's 4680 battery tray), and die-casting processes (such as integrated die-casting) simplify the welding process and reduce weight by 15%-20%.
Application of plastic composite materials: For example, glass fiber reinforced PA6 materials are used in non-load-bearing parts to further reduce weight, but the compatibility problem with the metal connection interface needs to be solved.
b. Lightweight design strategy
Topology optimization: Reduce redundant materials through CAE simulation and reduce the weight of the tray while ensuring strength.
Thin-wall and integrated: The wall thickness of the tray is reduced from 3mm to 1.5-2mm, and functional components such as BMS brackets and wiring harness channels are integrated to reduce the number of parts.
(4) Integration and modular design
a. High integration of functional components
CTP trays need to integrate modules such as battery management system (BMS), high-voltage connectors, and fireproof isolation layers.
b. Modular and compatible design
The welding production line needs to support mixed production of multiple models of trays, be able to achieve "one-click change of type", and be compatible with tray structures of different battery cell sizes (such as square and cylindrical).
2-Specific challenges of CTP technology innovation to welding process
CTP (Cell to Pack) technology has significantly improved space utilization and energy density, but it has also posed unprecedented challenges to welding process.
(1) The difficulty of controlling welding defects has increased sharply
a. Porosity and sealing challenges
After CTP technology eliminates the module, the battery tray needs to directly assume the sealing function. Welding pores (a common defect in aluminum alloy welding) will directly lead to the risk of coolant leakage or gas penetration.
b. Cracks and material compatibility
High zinc aluminum alloys (such as 7 series) are prone to cracks due to thermal stress during welding.
c. Gap and assembly error
The direct integration of multiple cells leads to an increase in the number of splicing points of the tray structure. The accumulation of assembly errors may cause the weld gap to exceed ±1mm.
(2) Process adaptation problems brought about by the upgrade of the material system
a. Welding challenges of lightweight materials
The CTP tray material has shifted from steel to aluminum alloy (6061-T6, 7075-T6), magnesium-aluminum alloy (30% weight reduction) and carbon fiber composite materials. Aluminum alloy welding needs to solve the problem of insufficient penetration caused by the difficult melting of the oxide film and high thermal conductivity.
b. Dissimilar material connection technology
When the tray integrates functional components such as liquid cooling plate (copper/aluminum) and fireproof layer (ceramic matrix composite material), brittle intermetallic compounds are prone to appear at the interface of dissimilar materials.
(3) Upgraded structural complexity and precision requirements
a. Deformation control of large-size thin-walled structures
The wall thickness of the CTP tray is reduced from 3mm to 1.5-2mm, and the sensitivity of welding thermal deformation increases sharply.
b. High-density solder joints and process efficiency
The number of solder joints on a single tray has increased from 2,000 in traditional modules to more than 5,000.
Figure 2- Battery tray welding
3-Upgrade of production process and quality control
CTP technology promotes the transformation of battery tray welding from "single process" to "multi-technology collaboration, intelligence, and greening". Manufacturers need to focus on three major directions:
Technology upgrade: break through the control of defects such as pores and cracks, and adapt to lightweight materials;
Intelligent transition: full process digitization and AI quality inspection to achieve high-precision production;
Ecological collaboration: jointly build technical standards with material suppliers, equipment suppliers, and OEMs.
We will regularly update you on technologies and information related to thermal design and lightweighting, sharing them for your reference. Thank you for your attention to Walmate.
Lightweight materials like aluminum alloys have become mainstream due to their superior performance. However, challenges such as heat input control, deformation suppression, and process stability in thin-plate welding pose significant hurdles for traditional welding technologies. Cold Metal Transfer (CMT) welding, with its advantages of low heat input, spatter-free transfer, and intelligent parameter control, offers an innovative solution for battery tray manufacturing.
This article delves into precision control strategies of CMT technology in thin-plate welding for battery trays, analyzing its adaptability, process challenges, and composite application scenarios, aiming to provide theoretical and practical guidance for efficient, high-quality production.
Figure 1: CMT Welding of 104S Energy Storage Battery Liquid Cooling Lower Enclosure
1-Battery Tray Welding Requirements and CMT Adaptability
CMT technology, with its low heat input, spatter-free transfer, and intelligent parameter control, perfectly aligns with the high precision, low deformation, and efficiency demands of battery tray welding.
(1)Core Process Requirements for Battery Tray Welding
a. Material Compatibility and Lightweighting Needs
Battery trays primarily use lightweight aluminum alloys (e.g., 6xxx series, 6061) or carbon fiber composites for high-end models, requiring high strength (60%–70% base material tensile strength) and low density (aluminum alloy: 2.7g/cm³).
Dissimilar Material Joining: For hybrid steel-aluminum structures, thermal expansion coefficient differences must be addressed to minimize deformation.
b. Weld Quality and Performance Metrics
Low Heat Input and Deformation Control: For thin plates (0.3–3mm), deformation must be ≤2mm. Long linear welds require segmented welding or anti-deformation design.
Sealing and Strength: Welds must be fully sealed to prevent electrolyte leakage and pass shear tests (e.g., T/CWAN 0027-2022 standards).
Porosity Control: Aluminum alloy welding is prone to porosity, requiring a porosity rate ≤0.5%.
c. Production Efficiency and Automation Requirements
Batch production demands a welding speed ≥7mm/s, reducing single-tray welding time to 5–10 minutes.
Automated workstations must support dual-station design (simultaneous assembly and welding) and multi-robot collaboration.
(2)Key Advantages of CMT for Battery Tray Welding
a. Precise Low Heat Input Control
CMT reduces heat input by 33% compared to traditional MIG welding by retracting the welding wire to cut off current during droplet short-circuiting, eliminating burn-through risks for ultra-thin plates (0.3mm).
Alternating cold-hot cycles (arc heating-droplet transfer-wire retraction) minimize heat accumulation, controlling deformation to ≤1.5mm (BYD and BAIC case studies).
b. Process Stability and Quality Enhancement
Spatter-Free Welding: Mechanical retraction eliminates droplet spatter, reducing rework.
Porosity Optimization: Using Ar+30%He shielding gas reduces porosity by 50% compared to pure Ar, with pore sizes ≤0.3mm.
High Gap Tolerance: Accommodates assembly gaps up to 1.5mm, lowering fixture precision requirements.
c. Automation Integration and Efficiency Gains
Dual-station workstations (e.g., Taixiang Tech designs) enable parallel welding and assembly, doubling efficiency.
Symmetric robot welding (dual-robot synchronization) with anti-deformation design reduces cycle time to ≤10 minutes.
2-Challenges in CMT Process for Battery Trays
Figure 2: CMT Welding Process Flow
(1)Material Properties and Weld Defect Control
a. Porosity Sensitivity in Aluminum Alloy Welding
Aluminum alloy trays (e.g., 6061, 6063) are prone to porosity due to rapid solidification and hydrogen solubility changes. Shielding gas composition is critical: pure Ar results in ~5% porosity, while Ar+30%He reduces porosity to ≤0.5%. Inductance adjustment (e.g., negative tuning) optimizes molten pool flow, minimizing pore size.
b. Hot Cracking and Composition Segregation
Segregation of Mg, Si, etc., in aluminum alloys can cause grain boundary embrittlement. While CMT’s low heat input reduces HAZ, precise control of welding speed and wire feed is needed to avoid insufficient penetration or localized overheating.
c. Metallurgical Compatibility in Dissimilar Material Welding
Interfaces in Al-steel or Al-composite joints (e.g., crash beams and enclosures) must mitigate brittle phases (e.g., FeAl₃) and Zn vapor interference.
(2)Process Parameter Optimization Challenges
a. Balancing Penetration and Heat Input
Welds must meet T/CWAN 0027 standards for penetration depth (≥0.8mm). CMT’s low heat input may lead to insufficient penetration, requiring arc length adjustment or pulsed current to enhance penetration.
b. Trade-off Between Speed and Stability
Automated lines demand speeds ≥1.2m/min, but high speeds risk arc instability or uneven droplet transfer.
c. Gap Bridging in Complex Welds
Trays often feature large gaps (0.5–1.5mm) or irregular joints (e.g., T-joints).
(3) Compatibility of structural design and manufacturing process
a. Thin plate welding deformation control
The wall thickness of aluminum alloy pallets is usually 2-3mm. The deformation of traditional MAG welding can reach 1.2mm, while CMT welding can reduce the deformation to less than 0.3mm through low heat input. However, it is necessary to cooperate with anti-deformation tooling design and robot symmetrical welding (double-station workstation) to further improve the accuracy.
b. Continuity and sealing of long welds
The length of the sealed weld of the battery tray can reach several meters, and arc breaking or molten pool fluctuations must be avoided. CMT technology ensures the uniformity of the weld through more than 70 arc reignition cycles per second, and the airtightness qualification rate can be increased to 99% with the laser tracking system.
c. Synergy of multi-process composite applications
High-end pallets often use CMT+FSW (friction stir welding) composite process: CMT is used for complex structures (such as the connection between the frame and the bottom plate). FSW is used in high-load areas (such as longitudinal beams) to improve strength. The matching problem of the connection parameters of the two processes (such as preheating temperature and post-weld heat treatment) needs to be solved.
3-Typical application scenarios of CMT process in battery tray manufacturing
(1) Connection of the main structure of the battery tray
a. Frame and bottom plate welding
CMT process is widely used in the connection between the frame and the bottom plate of aluminum alloy battery trays, especially for long welds and thin plates (2-3mm thickness)
b. Connection between beam and bottom plate
In the design of CTP battery trays, due to the reduced number of beams and complex structure, CMT process is used for: High-precision positioning welding: The local connection between the beam and the bottom plate (such as T-joint) needs to avoid insufficient penetration. CMT achieves stable penetration ≥ 0.8mm through digital arc length control (such as Fonis CMT Advanced technology). Multi-material adaptation: If the beam is made of aluminum-magnesium alloy (such as 6061) and the bottom plate is high-strength aluminum, CMT can reduce pores through Ar+He mixed gas protection, while adapting to the thermal conductivity differences of different materials.
(2) Welding of thin plates and complex geometric structures
a. Thin-wall aluminum alloy welding (2-3mm)
The lightweight demand for battery trays promotes the application of thin plates, but traditional MIG welding is prone to deformation. The advantages of the CMT process are:
b. Ultra-thin plate welding: Taixiang Automation uses CMT technology to achieve spatter-free welding of 0.3mm ultra-thin plates for battery tray edge sealing structures.
c. Bridging of special-shaped welds: For special-shaped structures such as internal reinforcement ribs and anti-collision beams of the tray, the CMT Gap Bridging mode can fill the 0.5-1.5mm gap through wire retraction and arc redirection to avoid unfused defects.
d. Welds with high sealing requirements: The sealing of the battery tray is directly related to battery safety. The CMT process ensures it in the following ways:
· Continuous long welds: Using more than 70 arc reignition cycles per second (such as Fronius LaserHybrid technology) to ensure the continuity of several meters of welds, with an airtight pass rate of 99%.
· Low heat input control: Compared with laser welding, CMT has lower heat input, which reduces the thermal impact of molten pool fluctuations on the sealant layer and is suitable for the glue coating process.
(3) Multi-process composite manufacturing scenario
a. CMT+FSW composite process
In high-end battery tray production lines, CMT is often coordinated with friction stir welding (FSW):
Division of labor and cooperation: CMT is used for flexible welding of complex structures (such as frames and special-shaped joints), and FSW is used for high-load areas (such as longitudinal beams) to improve strength. For example, Shanghai Weisheng's automated production line uses a combination of CMT+FSW+CNC to increase the tray production efficiency by 30%.
Process connection optimization: Huashu Jinming's production line adopts a modular design, and achieves seamless connection with FSW through preheating parameter matching (such as local heating to 150°C after CMT welding).
b. Combined with FDS/SPR riveting technology
In the second-generation CTP technology, CMT is coordinated with friction self-tightening (FDS) and self-piercing riveting (SPR) technology: Hybrid connection solution: For example, the load-bearing area of the frame and the bottom plate adopts FSW, while the detachable parts (such as water-cooling plates and insulation layers) are pre-positioned by CMT welding and then fixed by FDS riveting, taking into account both strength and maintenance convenience.
The explosive growth of new energy vehicles and energy storage has positioned battery tray welding technology at the core of manufacturing processes. Facing the dual challenges of aluminum alloy lightweighting and complex structures, this article delves into battery tray welding technologies, comparing the principles, performance metrics, and application scenarios of conventional fusion welding, friction stir welding (FSW), and laser welding. Through multi-dimensional analyses of heat-affected zones (HAZ), joint strength, corrosion resistance, and more, we present a comprehensive evaluation of the advantages and disadvantages of these welding technologies.
1-Technical Principle Comparison
a. Conventional Fusion Welding
Principle: Uses heat sources such as electric arcs or plasma arcs to locally melt the welding joint, forming a molten pool that solidifies into a weld upon cooling. Protective gases (e.g., CO₂, argon) or flux are required to prevent oxidation, and filler materials such as wires or rods may be added.
Characteristics: High molten pool temperatures and rapid cooling lead to coarse columnar grains. The heat-affected zone (HAZ) is wide, with insufficient metallurgical processes, resulting in defects like pores and cracks.
b. Friction Stir Welding (FSW)
Principle: Utilizes a high-speed rotating tool to generate frictional heat with the workpiece, bringing the material to a thermoplastic state. Mechanical stirring and plastic flow achieve solid-phase bonding without forming a molten pool or requiring filler materials.
Characteristics: Welding temperatures remain below 80% of the material’s melting point. Dynamic recrystallization forms fine-grained structures, resulting in dense, defect-free welds with low heat input and minimal deformation.
c. Laser Welding
Principle: A high-energy-density laser beam is focused on the workpiece surface. Heat conduction (power density <10⁵ W/cm²) or deep-penetration welding (power density ≥10⁵ W/cm², forming a keyhole effect) achieves material melting and bonding.
Characteristics: Extremely narrow HAZ, deep penetration, high welding speed, and precision capabilities. However, it is sensitive to material surface reflectivity and requires strict control of process parameters.
a- Conventional Fusion Welding b- Friction Stir Welding c- Laser Welding
Figure 1: Principles of Common Battery Tray Welding Technologies
2-Performance Metrics
a. Heat-Affected Zone (HAZ) Comparison
Key Analysis:
Conventional Fusion Welding: High heat input leads to wide HAZ, grain coarsening, and metallurgical defects (e.g., pores), significantly reducing material performance.
FSW: Solid-phase bonding avoids molten pools. HAZ is divided into TMAZ and HAZ, with coexisting fine grains (NZ) and localized deformation (TMAZ).
Laser Welding: Ultra-narrow HAZ (0.1–0.5 mm) due to high energy density and rapid cooling, but keyhole effects may impact microstructural uniformity.
b. Joint Strength Comparison
Key Analysis:
Conventional Fusion Welding: Rapid solidification causes coarse grains and defects, significantly reducing joint strength. For example, MIG-welded aluminum alloys exhibit only 72.8% of the base material’s tensile strength.
FSW: Dynamic recrystallization forms fine grains (NZ), but TMAZ grain deformation and HAZ phase dissolution may create weak zones.
Laser Welding: High cooling rates suppress grain coarsening, yielding weld strength close to the base material.
c. Corrosion Resistance Comparison
Key Analysis:
Conventional Fusion Welding: Coarse grains and defects lead to preferential corrosion in HAZ and fusion lines.
FSW: NZ exhibits superior corrosion resistance due to fine grains and homogenization, but HAZ grain coarsening and secondary phase precipitation (e.g., Fe-containing phases) may create corrosion-sensitive zones.
Laser Welding: Narrow HAZ and uniform microstructure reduce active corrosion sites, but surface oxidation layers must be managed for optimal corrosion resistance.
3-Application Scenarios
a. Conventional Fusion Welding
· Applicable Areas:
Frame-to-Baseplate Connections: Used for welding the main structure of battery trays, such as aluminum profiles in BYD and BAIC models.
Edge Beams and Small Component Repair Welding: Suitable for complex or spatially restricted areas (e.g., edge beams, stiffeners) requiring supplementary welding with other processes (e.g., FSW).
· Suitable Workpieces:
Thick plates (e.g., steel trays or thick aluminum plates).
Non-sealed auxiliary structures (e.g., battery pack corner fixtures).
· Suitable Materials:
Aluminum Alloys: 6xxx series (e.g., 6061-T6) for thick plates, though joint strength is low (70%–80% of base material).
Steels: Cost-effective but heavy for steel battery tray frames.
· Limitations: High heat input causes significant deformation, unsuitable for high-precision or thin-plate welding.
b-Friction Stir Welding (FSW)
· Applicable Areas:
Baseplate Splicing: Long-seam splicing for aluminum trays, e.g., integrated cooling channel structures (Guangdong Walmate Tech case).
High-Sealing Zones: Battery tray-to-enclosure joints (e.g., Geely and XPeng models use double-sided FSW for sealing).
Complex Profile Connections: T-joints or hollow profiles (self-supporting via dual-shoulder FSW).
· Suitable Workpieces:
Aluminum extruded profiles (e.g., 10mm-thick baseplates with 2mm wall double-layer designs).
Dissimilar material welding (Al/Cu, Al/Mg), requiring specialized process optimization.
· Suitable Materials:
Aluminum Alloys: 6xxx series (6061-T6, 6005A-T6, 6063-T6), achieving 80%–90% base material strength.
Magnesium Alloys: Lightweight trays (e.g., Ruixiang Tech case), but heat input must be controlled to prevent grain coarsening.
· Technical Advantages: Solid-phase bonding avoids fusion defects, ideal for lightweight and high-sealing applications.
c-Laser Welding
· Applicable Areas:
High-Strength Steel Tray Critical Welds: High-stress zones (e.g., battery tray-to-body connections).
Precision Seal Welding: Cover plate seal welding (up to 40 mm/s speed with minimal HAZ).
Thin-Plate Splicing: Efficient splicing for thin aluminum/steel plates (<3mm), minimizing deformation.
· Suitable Workpieces:
High-precision, automated production lines (e.g., robotic laser welding systems).
Complex geometries (e.g., curved seams) requiring dynamic positioning systems.
· Suitable Materials:
High-Strength Steels: Tensile strength ≥1000 MPa, retaining 95% of base material strength.
Aluminum Alloys: Require surface pretreatment (e.g., anodizing) to reduce reflectivity, increasing costs.
· Limitations: Multi-pass welding for thick plates (>8mm aluminum), lower efficiency than FSW.
We will regularly update you on technologies and information related to thermal design and lightweighting, sharing them for your reference. Thank you for your attention to Walmate.
As a front-line engineer at a battery tray manufacturer, I have participated in multiple new energy vehicle battery shell projects and have a deep understanding of the industry's game in the triangle relationship of "lightweight-safety-cost". This article will analyze the selection logic and industrialization challenges of the current technology route from three dimensions: material route, manufacturing process and future trends, combined with actual cases.
1-Material route: the trade-off between lightweight and cost
The choice of battery shell material directly affects the performance and economy of the whole vehicle. The current mainstream routes can be summarized into three categories: aluminum alloy, high-strength steel and composite materials, each with its own applicable scenarios.
a. Aluminum alloy route: the main force of lightweight
· Extruded aluminum profiles: BYD's battery shells of many models are made of extruded aluminum profiles, which achieve weight reduction by optimizing the cross-sectional shape and improve structural rigidity.
· Die-casting integration: Tesla's 4680 battery is combined with CTC technology to reduce weight by more than 50%, but the mold cost exceeds 200 million yuan, and an annual production of more than 500,000 pieces is required to dilute the cost, which is suitable for large-scale production of leading car companies.
· Composite aluminum: Porsche Taycan adopts an aluminum-carbon fiber hybrid structure, which further reduces weight by 15%, but the cost increases by 30%, which is limited to high-end models.
b. High-strength steel route: counterattack in the cost-sensitive market
Hot-formed steel (such as 22MnB5) has a yield strength of 1500MPa, a penetration rate of over 40% in commercial vehicles, and a single GWh cost that is 40% lower than aluminum, but the energy density is sacrificed by about 8%. Therefore, high-strength steel is generally used in models below 100,000 yuan, taking into account both cost and basic safety.
c. Composite materials: a trial of high-end
· SMC material: The upper shell of the battery pack uses glass fiber reinforced composite materials, which is 30% lighter than steel, but the impact resistance shortcomings need to be compensated by increasing the thickness (3mm+5mm reinforcement).
· Carbon fiber: The shell of the BMW i3 is 50% lighter than aluminum, but the cost is as high as 800 yuan/kg, and it is difficult to mass produce. It is currently only used for luxury models.
Practice summary: Material selection needs to match the model positioning. The mid-range market (200,000-300,000 yuan) is mainly aluminum-based materials, the low-end market relies on high-strength steel, and luxury models explore carbon fiber composite solutions.
2- Manufacturing process: the balance between efficiency and reliability
The manufacturing process of the battery shell directly affects the production efficiency and product reliability. The current mainstream technical routes include die casting, extrusion welding and structural integration technology.
a. Die casting vs. extrusion welding
· Extruded aluminum profiles (used by GM Bolt): The cost of a single piece is about 800 yuan, which is suitable for mass production, but the process is complicated.
· Die-cast aluminum (NIO ET5): The cost of a single piece is 1,500 yuan, but the production efficiency is increased by 5 times, which is suitable for rapid production demand.
· Friction stir welding (FSW): Compared with traditional arc welding, the deformation is reduced by 50%, and the fatigue resistance is improved by 30%, but the equipment investment needs to be increased by 40%, which is suitable for commercial vehicles with high life requirements.
b. Structural integration technology
· CTP modularization (CATL Kirin battery): The space utilization rate is increased from 72% to 85%, and the cost is reduced by 15-20%, but the thermal runaway protection design needs to be strengthened.
· CTC chassis integration (Tesla Model Y): 370 parts are reduced and the battery life is increased by 54%, but the maintenance cost increases by 300%, posing a challenge to the after-sales system.
Key data comparison
Production line experience: CTP technology is still the current mainstream due to its high compatibility; while CTC requires in-depth collaboration between car companies and battery manufacturers and is unlikely to be popularized in the short term.
3- Future trends: technology integration and intelligent upgrade
a. Material composite
Aluminum/carbon fiber hybrid shell (such as Porsche Taycan concept) can reduce weight by 15% and achieve 800MPa compressive strength, but the interface bonding strength needs to be greater than 25MPa (Toyota bZ4X mass production qualification rate is only 65%), and process stability needs to be broken through.
b. Functional integration innovation
· Liquid cooling plate and shell integration (GAC magazine battery): cooling contact area increased by 50%, temperature difference control <5℃, but aluminum-copper dissimilar welding porosity needs to be optimized by laser swing welding.
· Embedded fiber optic sensor (Continental Group solution): real-time monitoring of shell strain and temperature, BMS response speed increased by 30%, but the sensor durability problem needs to be solved.
c. Green circulation system
BMW's closed-loop recycling of recycled aluminum technology reduces carbon emissions by 60%, but the performance loss of recycled materials needs to be controlled within 10%. In addition, large thin-wall die casting (such as LK 9000T die casting machine) requires precise control of mold temperature difference (±5℃), and burr cleaning efficiency becomes a bottleneck for mass production.
Process breakthrough direction:
· Compression of composite material molding cycle (such as LGF-PP injection molding cycle needs to be shortened from 180 seconds to 90 seconds);
· Application of digital twin technology to reduce collision simulation error from ±20% to ±5%, improving design reliability.
4- Market stratification and industrialization outlook
Short term (1-3 years): CTP+ extruded aluminum profiles are still the mainstream, and CATL continues to lead with a 34% market share;
Medium term (5-10 years): CTC and carbon fiber are accelerating their penetration in the high-end market, and it is expected that carbon fiber will account for 25% in 2030;
Long-term goal: Energy density will break through 400Wh/kg, and at the same time, based on the national standard 30-minute thermal runaway protection, further improve safety redundancy.
5-Conclusion
The essence of choosing the technical route of battery housing is "scenario-based adaptation", which requires comprehensive consideration of the positioning of car companies, cost thresholds and supply chain maturity. As engineers, we must embrace innovation in materials and processes, and also focus on the feasibility of mass production, and find the optimal solution in the dynamic balance between lightweight, safety and cost. In the future, with the maturity of intelligent and green manufacturing technologies, battery housings will gradually shift from "passive protection" to "active safety", providing solid support for the full popularization of new energy vehicles.
We will regularly update you on technologies and information related to thermal design and lightweighting, sharing them for your reference. Thank you for your attention to Walmate.
In the actual production, assembly and use process, the insulation withstand voltage failure of battery trays often occurs, like a reef hidden in the rapid development of the new energy industry, threatening the driving safety of vehicles and the reliable operation of the energy storage system. This article attempts to analyze the typical failure modes based on manufacturing practice, in order to provide some superficial references for relevant practitioners and help industry colleagues jointly explore effective ways to improve the insulation withstand voltage performance of battery trays.
Part 3 -Common failure analysis
Figure 1 Insulation withstand voltage test
1- Analysis of common failure mechanisms
The application scenarios of battery trays for energy storage and new energy vehicles are different, resulting in both commonalities and differences in insulation withstand voltage requirements and failure conditions, as follows:
a. Differences
Dynamic load: New energy vehicles need to deal with high-frequency mechanical vibrations of 20-2000Hz, while energy storage systems mainly face long-term static deformation, with cumulative deformation time exceeding 10 years.
Voltage level: The insulation withstand voltage of new energy vehicle battery trays must reach 3000VDC and above (based on GB 38031), while energy storage systems require insulation withstand voltage of 4200VAC and above (based on IEC 61439).
Failure acceleration factor: New energy vehicles generate thermal stress due to more than 2000 charge and discharge cycles per year, which accelerates insulation failure; energy storage systems operate 24/7, with an average annual operating time of more than 8000 hours, resulting in electrochemical aging, which in turn accelerates insulation failure.
b. Common points
Both need to achieve early warning of insulation failure through insulation resistance monitoring (not less than 100MΩ in cold state and not less than 1MΩ/kV in hot state) and partial discharge control (less than 5pC).
2- Common manufacturing defects that cause insulation withstand voltage failure of battery trays
(1) Insulation withstand voltage construction stage
a. Potential failure and cause analysis
· Material defects:
Cause: Insulation material withstand voltage level is insufficient, aging due to moisture or surface contamination (oil stains, metal debris).
Performance: Low insulation resistance (<100MΩ), leakage current exceeds the standard or direct breakdown during withstand voltage test.
· Process problems:
Cause: Residual metal debris (rough welding/cutting process), uneven thickness of insulation coating or uncured.
Performance: Partial discharge, insulation layer damage causes short circuit.
· Environmental interference:
Cause: High temperature and high humidity cause material moisture absorption and chemical corrosion.
Performance: Insulation performance deteriorates with the environment, and condensed water causes creepage.
· Test errors:
Cause: Wrong test voltage/time setting (such as not in accordance with GB/T 38661 standard), poor grounding.
Performance: Misjudgment of qualified or overvoltage damage to components.
b. Response strategy
· Material optimization: Select moisture-proof materials (such as carbon fiber composite materials) with a withstand voltage of ≥1000V DC and apply anti-fouling coating.
· Process control: Use automated welding/spraying equipment and set up a clean workshop.
· Environmental management: Install temperature and humidity monitoring and pre-dry materials before construction.
· Test specifications: Segmented testing (500V pre-test + 1000V formal test), calibrate instruments and record data.
(2) Module installation stage
a. Analysis of potential failures and causes
· Assembly error:
Cause: The insulation liner is missing and the bolts are too tight and the insulation layer is damaged.
Performance: Low insulation resistance between the module and the shell, and withstand voltage breakdown.
· Mechanical damage
Cause: Scratches on handling tools, metal debris piercing the insulation film.
Performance: Local short circuit or sudden failure during operation.
· Design compatibility
Cause: Insufficient electrical clearance due to size deviation between the module and the tray.
Performance: Parasitic capacitance causes voltage superposition breakdown.
· Electrical connection hazards:
Cause: Wear and failure of connector seal due to unfixed wiring harness.
Performance: Wear and leakage of high-voltage wiring harness insulation layer.
b. Countermeasures
· Error-proof design: Use positioning pin tooling to prevent missing installation, and customize bolt length to prevent over-tightening.
· Clean operation: Vacuum cleaning before installation, and wrap tools with anti-scratch silicone.
· Tolerance simulation: Verify the matching degree between the module and the tray through CAE to ensure that the gap is ≥10mm.
· Process inspection: After installation, perform random inspection with a megohmmeter (≥100MΩ) and perform segmented withstand voltage test to locate the fault point.
(3) System Integration Phase
a. Potential Failure and Cause Analysis
· Cross-system interface failure:
Cause: Component insulation parameters do not match (such as connector withstand voltage rating differences).
Performance: High-voltage busbar connection breakdown or BMS interference.
· Parasitic capacitance superposition:
Cause: The total parasitic capacitance increases after multiple modules are connected in parallel, and the capacitance rise effect is significant.
Performance: System-level test leakage current exceeds the standard.
· Environmental stress failure:
Cause: Vibration causes insulation fatigue and coolant seeps into the high-voltage interface.
Performance: Insulation resistance decreases periodically during operation.
· Test blind spot:
Cause: Failure to simulate real working conditions (high temperature/vibration/humidity).
Performance: Passed in the laboratory but failed in actual operation.
b. Countermeasures
· Compatibility design: Unify supplier component standards.
· Parasitic capacitance control: Add polyimide isolation layer and optimize layout through SPICE simulation.
· Working condition simulation test: perform "-40℃~85℃ cycle + 5Hz~200Hz vibration + withstand voltage" composite test.
· Intelligent monitoring: integrated online insulation monitoring module, AI predicts potential risks.
3-Insulation withstand voltage test
Insulation withstand voltage test is both a safety measure and a possible cause of failure. The insulation withstand voltage test itself may cause failure due to improper operation, equipment problems or design defects, and even cause secondary damage to the device under test.
(1) Common types of failure during the test
(2) The response strategies are as follows:
a. Precise control of test parameters
· Voltage and time: set according to standards; enable dynamic compensation algorithm for capacitive loads (such as battery modules) to suppress the capacity increase effect.
· Leakage current threshold: set according to equipment specifications (such as ≤10mA) and distinguish between DC/AC modes (DC testing requires a lower threshold).
b. Equipment and operation specifications
· Instrument calibration: The withstand voltage tester is calibrated every 6 months, and the high-voltage line insulation layer is visually inspected daily.
· Safe operation: forced disconnection of low-voltage circuits before testing; use robotic arms or automatic fixtures to avoid manual contact with high-voltage areas.
c. Failure prevention and repair
· Segmented testing: test complex systems in modules (such as testing the high-voltage bus first, then testing the module insulation) to locate the breakdown point.
· Failure repair: use epoxy resin to fill the damaged area after breakdown, and after repair, it is necessary to pass the full voltage gradient test again (such as 500V→1000V step pressure).
d. Environment and data management
· Environmental control: Start the dehumidifier when the humidity in the test area is greater than 75%, or delay the test until the environment meets the standard.
· Data traceability: Record the test voltage, leakage current curve, and environmental parameters, and use the MES system to associate the production batch number.
We will regularly update you on technologies and information related to thermal design and lightweighting, sharing them for your reference. Thank you for your attention to Walmate.
The battery tray uses insulating materials mainly to prevent current leakage, protect personnel safety, and ensure the normal operation of the battery system. When selecting, it is necessary to consider the material's insulation properties, heat resistance, chemical stability and mechanical strength. These factors together determine the application effect of the insulation material in the battery tray, thus affecting the safety and reliability of the entire battery system.
Part 2 - Pressure resistance performance guarantee
Figure 1 Electric vehicle battery tray
1-Insulation material selection and solution design
In the selection of insulation materials for battery trays, the focus is on the dielectric properties, environmental tolerance and mechanical properties of the materials. The following are 6 commonly used insulation materials in battery trays and their related information:
When designing a solution, we generally need to comprehensively consider the three key factors of application scenario, performance requirements and cost budget to select the appropriate insulation material. For example:
(1) Select according to the application scenario: In a high-voltage, high-current energy storage battery system, if the battery tray side requires high-performance insulation material, it is recommended to use the PI film attachment solution; if the insulation performance requirements are not high, the insulation powder spraying solution can be selected. For the battery tray bottom plate, if a low-cost insulation solution is sought, the insulation powder spraying or insulation paint coating solution is more suitable.
(2) Select according to performance requirements: When the requirements for insulation performance, high temperature resistance, chemical corrosion resistance, etc. are high, the PI film attachment solution is a better choice; if these performance requirements are relatively low, the insulation powder spraying or insulation paint coating solution can meet the needs.
(3) Select according to the cost budget: When the cost budget is limited, the insulation powder spraying or insulation paint coating solution is more economical; when the cost budget is sufficient, the PI film attachment solution can be selected.
2-Technical requirements for PI film for battery trays
(1) Material: PI, base film thickness 0.1-0.14mm, back adhesive thickness 0.03mm, PI film thermal conductivity > 0.3W/(m·k);
(2) Pressure resistance: AC 3000V, 60S, leakage current ≤ 0.5mA;
(3) (cold paste) 180° peel strength ≥ 15N/24mm;
(4) Insulation: DC 1500V, 60S, insulation resistance > 1000MΩ;
(5) Heat and electrolyte resistance insulation performance: at 500℃, 700V DC voltage, add 2ml electrolyte (test area 13000mm<H>2<H>), keep for 1h, no breakdown and no sparking;
(6) Heat and insulation performance: at 500±2℃, continue baking (muffle furnace) for 0.5h, the overall morphology of the sample changes without spontaneous combustion, and the sample passes twice. AC 1000V is applied to the surface, and the voltage is increased from 0V to 3000 for 10S, and then continued for 60S. The tested sample has no breakdown and no sparking;
(7) Flame retardant grade: UL94 V-0;
(8) High temperature and high humidity: After 1000 hours of 85℃ and 85% humidity test, the sample has no cracking and no deformation, meeting the national standard "GBT 13542.6-2006 Electrical insulation film Part 6: Polyimide film for electrical insulation" on insulation resistance, withstand voltage value, and electrical strength requirements. The tensile strength, elongation at break, and peel strength are reduced by less than 30% compared with the unaged material;
(9) Hot and cold shock: 85℃ for one hour, switched to -40℃ for one hour, and then switched to 85℃ for high and low temperature cycles. The switching time is included in one hour, and the switching time is ≤3min. After 1000 hours of high and low temperature cycling, the sample has no cracking and deformation, meeting the national standard "GBT 13542.6-2006 Electrical insulation film Part 6: Polyimide film for electrical insulation" for insulation resistance, withstand voltage value, and electrical strength requirements. The tensile strength, elongation at break, and peel strength are reduced by less than 30% compared to the unaged material.
(10) Salt spray test: Neutral salt spray test (NSS) test in GB/T 10125 standard, temperature 35℃, humidity ≥85%RH, test solution is (5±0.1%) (mass fraction) NaCl, PH=6.5~7.2, continuous spraying for 72h. After the salt spray test, the sample has no cracking and no deformation, meeting the national standard "GBT 13542.6-2006 Electrical insulation film Part 6: Polyimide film for electrical insulation" for insulation resistance, withstand voltage value, and electrical strength requirements. The tensile strength, elongation at break, and peel strength are reduced by less than 30% compared with the unaged material.
(11) The product complies with RoHS.
Figure 2 PI film for battery tray
3-Insulation withstand voltage test methods and solutions to common problems
(1) Insulation withstand voltage test method
Insulation withstand voltage test is an important means to evaluate the insulation performance of electrical equipment, mainly including DC withstand voltage test and AC withstand voltage test. DC withstand voltage test detects whether the insulation material breaks down within a specified time by applying DC high voltage. The equipment includes DC high voltage generator, voltmeter, etc. The steps are applying voltage, maintaining voltage and reducing voltage. AC withstand voltage test applies AC high voltage. The equipment and steps are similar to DC test. Both methods require preparation before testing to ensure that the equipment is well grounded and the surface condition of the insulation material is closely observed during the test.
(2) Common problems and solutions
Common problems in insulation withstand voltage test include insulation breakdown, excessive leakage current and test equipment failure. Insulation breakdown may be caused by insufficient insulation material performance, internal defects or moisture. Solutions include selecting high-quality materials, strengthening maintenance and improving insulation structure. Excessive leakage current may be caused by reduced insulation resistance, surface contamination or insufficient accuracy of test equipment. Solutions include keeping the insulation material clean and dry and calibrating the test equipment. Test equipment failure may be caused by equipment aging, improper maintenance or improper operation. Solutions include regular maintenance, correct operation and timely repair.
We will regularly update you on technologies and information related to thermal design and lightweighting, sharing them for your reference. Thank you for your attention to Walmate.
With the rapid development of new energy vehicles and energy storage technologies, the safety and reliability of battery systems, as core energy carriers, have attracted much attention. As the supporting and protective structure of the battery module, the electrical insulation performance of the battery tray is directly related to the safe operation of the vehicle, battery life and personal safety of the user. The electrical safety design of the battery tray is the cornerstone of battery system safety. Through multi-level protection, such as insulation, structure, heat, and monitoring, it ensures stable operation of the battery under complex working conditions, reduces the risk of fire, explosion, or electric shock caused by electrical failure, and extends battery life and improves system reliability.
This article focuses on the electrical insulation safety of battery trays, systematically explains its design principles, the verification method of pressure resistance performance, and the root cause analysis and improvement strategies of typical failure cases, in order to provide theoretical support and practical reference for the high-safety design of battery systems.
Previous article - Design points
The core of the electrical safety design of the battery system lies in the trinity of "prevention-control-emergency": prevent failures through insulation isolation, reliable connection, thermal management and other measures; achieve real-time control with the help of sensors and BMS; use fire prevention, pressure relief and other designs to deal with extreme situations. All key points need to work together to ensure the safety and reliability of the battery system throughout its life cycle, while taking into account maintainability and compliance. The battery tray is not only a structural support in the battery system, but also has multiple functions such as electrical isolation, thermal management, anti-slip, mechanical protection, grounding and modular design, which is crucial to ensuring the electrical safety of the battery system.
1-Structural support ensures electrical connection reliability
The battery tray ensures the reliability and safety of electrical connections under complex working conditions by fixing battery modules, reducing mechanical stress, resisting vibration and shock, maintaining alignment, and integrating environmental protection and thermal management.
Figure 1 Battery tray
A.Physical support and fixation ensure the precise alignment of electrical connection points (such as busbars, wiring harnesses, and connectors) to avoid poor contact caused by structural deformation or displacement; provide a rigid frame to fix battery modules and connectors to prevent loosening or breakage caused by vibration or impact.
B.Environmental isolation and protection: prevent the intrusion of water vapor, dust, salt spray, etc. through sealing design (such as IP67/IP68), avoid short circuits caused by corrosion or insulation failure; block external mechanical shock or foreign body impact to protect high-voltage connection components.
C.Thermal management synergy: integrated heat dissipation structure (such as liquid cooling plate, thermal pad) balances temperature to prevent local overheating from causing oxidation or welding of connection points; reduce thermal interference between adjacent modules through thermal insulation design to avoid material expansion differences caused by temperature gradients.
D.Electromagnetic compatibility (EMC) support: suppress electromagnetic interference through metal shielding layer or conductive coating to protect low-voltage signal lines (such as BMS communication lines) from high-voltage circuit interference.
2-Isolation protection builds an efficient isolation environment
The focus of battery electrical isolation design is to create an environment that can effectively isolate high voltage electricity and ensure efficient operation of the system, ensuring that the battery module can be safely packaged under any operating conditions, preventing accidental release of electrical energy, and thus avoiding potential electrical risks.
A.The battery tray structure takes into account both load-bearing and isolation protection:
l Aluminum alloy materials, such as extruded aluminum alloy, are preferred to achieve lightweight while maintaining high rigidity and impact resistance. The outer frame is used to bear the weight of the entire battery system and external impact. Closed-section profiles are used to enhance structural strength. The inner frame is designed to support battery modules and water-cooled plates, etc., to ensure their stability and heat dissipation requirements.
l Insulating materials are used as pads or coatings to ensure good electrical isolation between the battery module and the tray. The high-voltage wiring harness should have a dedicated management path and insulating sheath to ensure electrical clearance and creepage distance with the tray.
l Advanced welding technologies such as stir friction welding are used to improve connection strength while reducing heat-affected zones, avoiding deformation and potential cracks. For parts that are inconvenient to weld, bolt connections or riveting are used, combined with sealants, to ensure the reliability of mechanical connections and electrical isolation.
l Modularity is considered during design to facilitate battery replacement and maintenance without affecting the stability of the overall structure.
Figure 2 Schematic diagram of electrical clearance and creepage distance
B. Key points of high and low voltage isolation design:
l The positive and negative points of the battery system must be isolated from the low-voltage power supply system and the battery tray to ensure that there is sufficient electrical clearance and creepage distance between the high-voltage circuit and the low-voltage control circuit to meet safety standards and prevent high voltage leakage to the low-voltage system.
l The high-low voltage isolation design needs to consider electromagnetic compatibility (EMC) to ensure that the isolation measures will not introduce interference and keep the system running stably.
l High-impedance connection, the high and low systems are connected through high-impedance, and only the vehicle body ground (battery tray) is allowed to limit the flow of current to ensure that the high-voltage system fault spreads to the low-voltage system.
l Physical isolation measures, when designing the battery tray, the high-voltage and low-voltage components can be arranged in different closed chambers to reduce mutual influence through physical separation; use insulating materials as pads between the battery module and the tray, such as polymer plastics or rubber, to ensure physical and electrical isolation.
l Consider the maintainability of the isolation measures during design to ensure that they can be repaired or replaced safely when necessary.
C.Key points of contact protection design:
l High-voltage harness management: High-voltage harnesses should be properly wrapped in insulating sheaths and managed in an orderly manner through fixing clips or wire troughs to avoid exposure and reduce the risk of direct contact.
l Safety partitions: Insulating partitions are set between battery modules and between battery modules and tray walls to prevent the risk of indirect electric shock caused by electrolyte leakage when the battery is damaged.
l High-voltage component packaging: Key components such as high-voltage connectors and relays are packaged to ensure that these components will not be directly touched by accident even inside the tray.
l Enclosed design: The battery tray is designed as a closed structure as a whole, using a metal or composite shell to ensure that the internal high-voltage components are not exposed, and the shell itself must also have good insulation properties.
l Locking mechanism: For maintainable high-voltage connection points, a locking mechanism is used to ensure that they will not be easily opened during non-professional operations, reducing the risk of accidental contact.
l Insulating material application: Insulating materials are used as an isolation layer between the battery tray and the battery module to ensure that even if the tray is damaged, the human body can be prevented from directly contacting the live parts. These materials include but are not limited to polymer plastics, rubber gaskets or coatings.
3-Electrical Logo Design
The electrical identification of the battery tray can not only improve the safety of operation, but also simplify the maintenance process and reduce the risk of incorrect operation.
A.Clear identification
l Clearly mark warning signs such as "High Voltage Danger" and "Do Not Touch" on the battery tray and surrounding high-voltage components to ensure quick identification even in an emergency.
l Use internationally accepted color coding, such as red or orange to mark high-voltage areas and blue for direct current, to intuitively distinguish different electrical characteristics.
l Apply standardized electrical safety symbols, such as the graphic symbols in IEC 60417, to indicate high voltage, grounding, power-off points, etc. to ensure global understandability of information.
l Include the serial number, production date and batch information of the battery tray for easy tracking and recall management.
l Select wear-resistant and corrosion-resistant materials and printing technologies to ensure that the label remains clear and readable throughout the life cycle of the battery tray.
B.Warning signs
l High voltage hazards are clearly marked on the battery tray and surrounding areas to remind maintenance personnel to pay attention to electrical safety and comply with operating procedures.
l Indicate safe operating distances, especially near high-voltage connectors and exposed locations, to remind people to keep an appropriate distance.
C.Operation guide
l Clearly mark the grounding locations of the battery system and the tray to ensure proper implementation of grounding measures.
l Identify safe test points and maintenance access points, which should be designed to operate at low voltage or non-powered conditions.
l Basic operating and safety instructions can be briefly listed in non-critical areas of the tray to guide the correct operating process.
We will regularly update you on technologies and information related to thermal design and lightweighting, sharing them for your reference. Thank you for your attention to Walmate.
The most popular water blocks are basically pure copper microchannel type. The copper bottom plate is directly processed with skived, and the fins are integrated with the bottom plate to reduce thermal resistance. The bottom plate and the cover plate are brazed or diffused to ensure the reliability of the seal.
1- Advantages of using skiving process to manufacture water blocks
l Integrated design: The skiving process can integrate the floor and fins, which is helpful to reduce contact thermal resistance and improve thermal conductivity. In addition, the integrated bottom plate and fin design can also improve structural strength.
l High-precision processing: The skiving process can produce a very fine tooth structure, and the tooth height, tooth thickness and tooth pitch can be precisely controlled, which makes the heat sink fins denser, the heat dissipation area larger, and the heat dissipation more efficient. At the same time, it can also better meet the personalized needs of different customers for shape, size, etc.
l The production efficiency is high, and the skiving process can be mass-produced. Compared with traditional CNC, the skiving process can process multiple gear pieces at the same time, which greatly improves the production efficiency.
Figure 1: Water block base with different processing technologies a-Skiving b-CNC c-Cold Forging
2- Cost structure of skiving water block
l Design and development costs: The design complexity of the skiving water blockis relatively high, especially when high heat dissipation performance requirements are required, which requires complex process design and optimization.
l Material cost: The materials used in the skiving process are mainly aluminum and copper alloy. The design of combining aluminum plate and copper alloy is more common in radiator manufacturing and has a higher cost performance, so the quality of aluminum and copper directly affects the cost.
l Processing cost:
Skiving process cost: The skiving process involves high-precision CNC machining equipment, such as three-axis CNC machine tools. The use of such equipment requires a high investment cost, and the technical requirements for operators are also high, which will increase production costs.
Welding process cost: In the manufacturing process of water blocks, welding process is also an important cost factor. Vacuum brazing and diffusion welding are two commonly used welding methods. Brazing can weld multiple joints at the same time, has high production efficiency, requires brazing materials, has high requirements for process conditions, and is difficult to control quality; diffusion welding equipment has a large one-time investment, and diffusion welding does not require fillers, but has high requirements for the surface processing of the workpiece.
Surface treatment cost: Common surface treatment methods include anodizing, plating, etc. For aluminum alloy water blocks, anodizing can improve surface hardness, wear resistance and corrosion resistance, while increasing the aesthetics of the product; copper water blocks will be plated, such as nickel plating, to prevent copper oxidation and corrosion, and increase product life and reliability.
l Other costs: inspection and testing, packaging and transportation, etc.
3-Cost optimization suggestions
l Optimizing material usage
Choose the right material: For example, copper has high thermal conductivity, high unit density, and high unit price; aluminum has light weight, low unit price, and slightly poor thermal conductivity.
Material usage: Ensure efficient use of materials and reduce material waste by accurately calculating material thickness and margin.
Figure 2: Schematic diagram of material consumption calculation
l Optimizing production processes
Simplify molds: Design simple molds to reduce mold complexity and cost.
Reduce scrap rate: Reduce scrap rate through precise process control and quality inspection.
One-step molding: Optimize process routes, reduce multiple processing steps, and improve production efficiency.
We will regularly update you on technologies and information related to thermal design and lightweighting, sharing them for your reference.Thank you for your attention to Walmate.
As a key component of the liquid cooling system, the design of the water block must take into account multiple factors such as heat exchange performance, structural strength, corrosion resistance, leakage resistance, and cost control. The water block is usually designed with a complex heat exchange slot structure (i.e., flow channel), and the quality of its internal flow channel design directly determines the heat exchange efficiency of the entire system.
Part 2:Water block design, processing technology and challenges
1-Design requirements for water blocks in different scenarios
l High Performance Computing:
High-performance computing devices (such as high-performance CPUs, GPUs, etc.) generate a lot of heat during operation, so the water block needs to have efficient heat dissipation capabilities. In order to meet the high heat flux density heat dissipation requirements, the water block usually adopts a high-density microchannel design to increase the heat exchange area and improve the heat dissipation efficiency. In addition, some designs integrate the water block directly onto the CPU, eliminating the step of applying silicone grease, which not only simplifies the assembly process, but also further improves the heat dissipation performance. In terms of reliability, the water block must have excellent sealing performance to prevent leakage and ensure long-term stable operation.
l Graphics card cooling:
Graphics cards are high heat generating areas, so the water block needs to have a full coverage design to ensure that all heat generating components on the graphics card can be effectively cooled. At the same time, graphics card cooling requires a high flow rate of coolant, so the internal structure of the water block needs to support high flow rate to quickly remove heat.
l Data Center:
In data centers, the structural design of water block needs to meet multiple requirements such as efficient heat dissipation, low noise, high reliability, adaptability to high power density, intelligent management and environmental adaptability to ensure stable operation and efficient heat dissipation of data centers.
2- Evolution trend of water block structure
The evolution trend of water block structural design reflects the dual pursuit of technological innovation and performance improvement, which is mainly reflected in the following aspects:
l Improved heat dissipation performance:
Increase contact area: Some water block designs improve heat dissipation performance by increasing the contact area with the heating element. For example, a large-area copper base design can achieve good contact and heat conduction.
Optimize the internal structure: Optimize the internal water channel. One idea is to optimize the fluid flow, such as changing from ordinary fins to steering fins, presenting a long strip flow channel, promoting flow boundary layer separation, reducing boundary layer thickness, and improving heat exchange efficiency; another idea is to increase the heat capacity area, such as changing from traditional coarse water channels to microchannel design, which significantly increases the contact area between the coolant and the base plate and enhances the heat dissipation efficiency. In some designs, the coolant is sprayed onto the microchannel base plate through a guide plate to increase the local flow velocity and turbulence, greatly improving the heat absorption efficiency.
l Integrated and intelligent design:
Integrated design: The integrated design integrates the water pump, heat sink fins, heat conduction base and other components together to reduce the number of connection points and improve system stability and heat dissipation efficiency.
Multifunctional integration: In addition to heat dissipation performance, modern water blocks also have temperature display and monitoring functions.
Modular design: The modular buckle structure improves the convenience and freedom of organization.
Figure 1: Water block base with different fin thicknesses
l High-performance materials and finishes:
The use of high-performance materials such as pure copper base, combined with surface treatment technologies such as nickel plating, improves thermal conductivity and corrosion resistance.
3-Processing technology and challenges
l Material properties affect processing:
Material hardness and toughness issues: Radiators made of different materials, such as copper, aluminum and their alloys, have different hardness and toughness, and different requirements for processing tools and processes. Materials with higher hardness will wear faster and require more frequent tool changes; materials with better toughness are prone to deformation and burrs during cutting.
The processing of copper-aluminum composite materials is complex: copper-aluminum composite material shovel-tooth heat sinks must first be made into composite materials using continuous casting semi-molten state pressing technology, and then shovel-tooth processing is performed. The process is more complicated and requires higher precision for equipment and processes.
l High dimensional accuracy requirements
It is difficult to ensure the consistency of tooth height and thickness: For some high-density tooth heat sinks, the height and thickness of each tooth are required to be highly consistent to ensure the performance and uniformity of the heat sink. If the difference in tooth height and tooth thickness is too large, it will lead to uneven heat transfer and affect the heat dissipation effect. During the processing, high-precision equipment and automated control systems are required to ensure that the specifications of each tooth are consistent.
It is difficult to control the tooth spacing: When the teeth on the heat sink are too dense, their density and spacing make the processing process more complicated, and the processing equipment needs to have higher speed and precision to maintain the uniformity of the teeth. For example, when the tooth spacing is too small, the tool is prone to interference during cutting, affecting the processing accuracy and surface quality.
l Strict surface quality requirements
Burr problem: Burrs are easily generated during the processing, which not only affects the aesthetics of the radiator, but also may hinder air flow and reduce the heat dissipation effect. The generation of burrs may be caused by low material cutting accuracy, wear of processing tools, etc., and corresponding deburring processes need to be adopted to solve them.
Surface roughness: The surface roughness of the radiator affects its heat dissipation performance and subsequent surface treatment effects. Excessive surface roughness will increase the resistance to air flow and reduce heat dissipation efficiency. Additional surface treatment is required to reduce the roughness, which increases processing costs and time.
l High processing equipment and process requirements
Equipment accuracy and stability: Gear shoveling requires a high-precision gear shoveling machine, and the accuracy of the equipment directly affects the dimensional accuracy and surface quality of the teeth. At the same time, the equipment must have good stability to ensure dimensional consistency during long-term processing.
Tool selection and wear: Suitable tools are crucial to processing quality. The material, geometric parameters, etc. of the tool need to be selected according to the material properties. During the processing process, tool wear will lead to increased cutting force, decreased dimensional accuracy and increased surface roughness, and the tool needs to be adjusted or replaced in time.
Feed speed and cutting depth: Unreasonable settings of feed speed and cutting depth can easily lead to processing defects. If the feed speed is too fast and the cutting depth is too large, the tool will be overloaded, resulting in tool snatching, tool bounce, tool drop, etc., affecting processing accuracy and surface quality.
Figure 2: Skiving process
l High customization requirements
Different application scenarios have different requirements for the size, shape, tooth height, tooth thickness, tooth spacing and other parameters of the radiator, which need to be customized according to specific needs. This requires the processing manufacturer to have flexible process adjustment capabilities and rich experience to meet diverse customization needs.
We will regularly update you on technologies and information related to thermal design and lightweighting, sharing them for your reference.Thank you for your attention to Walmate.
With the rapid growth of global artificial intelligence computing power and the continuous increase in chip thermal design power consumption (TDP), the cooling demand of data centers is facing unprecedented challenges. At the same time, the increasingly stringent energy-saving and carbon-reduction policies around the world have further promoted the innovation of cooling technology. In this context, traditional air cooling solutions have gradually become difficult to meet the dual requirements of efficient heat dissipation and energy saving. Liquid cooling technology is rapidly rising due to its excellent heat dissipation performance and significant energy-saving advantages, becoming the mainstream choice for data center cooling solutions. As the core equipment for data processing and storage, the performance and stability of the server are directly related to the operating efficiency of the entire system. The core components of the server - including the motherboard, CPU, memory, hard disk and graphics card - will generate a lot of heat under continuous high-load operation. If the heat cannot be dissipated in a timely and effective manner, it will seriously affect the performance and life of the server. To this end, advanced liquid cooling systems have been introduced into server cooling solutions and installed directly on major heat sources such as CPUs and graphics cards to significantly improve heat dissipation efficiency.
Part 1:Water Block Structure and Working Principle
One of the core components of the liquid cooling system is the water block, which is usually made of copper or aluminum materials with high thermal conductivity and is designed with precise water channels and heat sink structures. These water blocks fit tightly to the surface of heat sources such as CPUs and GPUs, and quickly absorb and transfer heat through the cooling water circulating inside. The heat is then transported to the heat sink, which is then circulated through the water cooling system and eventually dissipated into the surrounding air.
Figure 1: Chip thermal power consumption trends of mainstream chip manufacturers
1- Common water block types and characteristics
l Microchannel water block
Features: The microchannel water block adopts a precise micro-water channel design. The water channel structure is fine and complex, which can significantly increase the contact area between the coolant and the heat-generating components, thereby greatly improving the heat dissipation efficiency. The micro-water channel design can also produce a strong turbulence effect during the flow of the coolant, further enhancing the convection heat transfer coefficient and achieving efficient heat transfer.
Application scenarios: It is particularly suitable for CPUs and GPUs with high heat generation, especially in high-performance computing, overclocking, and data centers, which have extremely high heat dissipation requirements.
l Large flow water block
Features: The internal structure of the large-flow water block is relatively simple, usually using a copper plate or etched groove design, and the manufacturing cost is low. Its core advantage is that it relies on high-speed water flow to quickly remove heat, and is suitable for use with a large-flow water cooling system. Although the structure is simple, its efficient heat dissipation capacity makes it a very cost-effective choice.
Application scenario: Suitable for scenarios with certain requirements for heat dissipation efficiency but limited budget, such as mid-to-high-end DIY computer systems or small and medium-sized server clusters.
l Injection type water block
Features: The jet-type water-cooling block sprays the coolant from a narrow nozzle to the micro-channel bottom plate at high speed through the guide plate, forming a strong turbulence effect and significantly improving the heat dissipation efficiency. This design not only increases the contact area between the coolant and the base, but also further optimizes the heat exchange performance through high-speed flow.
Application scenarios: Suitable for high-performance CPUs and GPUs, especially in high-heat dissipation requirements and high-flow scenarios, such as overclocking computing, artificial intelligence training, and graphics rendering.
2-General structure of water block
A water block is a metal block with water channels inside, usually made of copper or aluminum. It does not come into contact with the CPU, graphics card or other heat generating devices. Its structural design directly determines the quality of heat dissipation performance. A typical water block usually consists of the following key parts:
l The base is usually made of high thermal conductivity materials such as copper or aluminum alloy, and the surface is finely processed to ensure close contact with the heat-generating components. The base is equipped with a complex flow channel design to increase the contact area between the coolant and the base.
l The cover plate, together with the base, forms a flow channel sealing cavity to protect the flow channel, seal and other components inside the water block from dust, impurities and external physical damage.
l The water inlet and outlet are the interfaces for the coolant to enter and exit the water cooling head. They are generally designed on the side or top of the water cooling head to ensure that the coolant can flow in and out smoothly. The position design needs to consider the flow path of the fluid to reduce flow resistance and increase the flow rate of the coolant.
l Clips are used to firmly fix the water cooling head to the CPU or other heat-generating components to ensure a tight foundation and achieve efficient heat conduction.
Figure 2: Typical structure of water block
3- Comprehensive optimization of heat dissipation performance
l Flow channel design optimization
Increase the contact area of the flow channel: By designing narrower and denser flow channels, the contact area between the coolant and the base can be significantly increased, thereby improving the convective heat transfer coefficient. For example, the microchannel design has a fine water channel structure. The design parameters of the microchannel water block (such as channel width, height, and spacing) have a decisive influence on its heat dissipation efficiency: as the channel width decreases, the heat transfer coefficient increases significantly, thanks to the enhanced turbulence effect of the coolant flow in the narrow channel, thereby improving the heat exchange efficiency; the higher channel height helps to increase the flow space of the coolant, thereby improving the heat transfer performance; the smaller channel spacing can increase the contact area between the coolant and the heat source, thereby improving the heat dissipation efficiency.
It can also be optimized by optimizing the flow channel layout and reducing the flow channel bends: a reasonable flow channel layout can ensure that the coolant evenly covers the surface of the heat-generating components and reduce the local temperature difference. The bends in the flow channel will increase the head loss and internal flow resistance, thereby reducing the cooling efficiency. The number of bends should be minimized in the design. If it cannot be avoided, the bends should be designed as smooth transitions to reduce the pressure drop and optimize the flow performance.
l High thermal conductivity materials: The base of the water block is usually made of high thermal conductivity materials such as pure copper or aluminum alloy. These materials can efficiently transfer heat from the heat source to the coolant, thereby improving the overall heat dissipation performance.
l Structural parameter optimization, such as base substrate thickness, fins, spoiler structure, etc. The increase in substrate thickness will lead to an increase in the maximum temperature, so the design needs to find the best balance between heat dissipation effect and structural strength; by increasing the height, thickness and spacing of the fins, the heat dissipation performance can be improved, but at the same time, the flow resistance will also increase. Find the best fin design combination to maximize the heat dissipation performance. The shape of the spoiler column can effectively enhance the turbulence effect and improve the heat exchange efficiency.
We will regularly update you on technologies and information related to thermal design and lightweighting, sharing them for your reference.Thank you for your attention to Walmate.
Based on actual production, this article summarizes the common problems, causes and improvement methods in the stir friction welding process for your reference.
1-Surface defects
(1) Surface grooves
l Problem description: Surface grooves, also known as plowing defects, usually appear on the upper surface of the weld, tending towards the advancing edge of the weld, and are groove-shaped.
l Cause: The thermoplastic metal flow around the weld is insufficient, and the plastic metal of the weld cannot fully fill the instantaneous cavity left by the stirring needle during its movement.
l Improvement method: Increase the shoulder diameter, increase the pressure, and reduce the welding speed.
Figure 1: Grooves
(2)Edge burrs
l Problem description: Flash burrs appear on the outer edge of the weld and are wavy in shape.
l Cause: Improper matching of rotation speed and welding speed, excessive downward pressure.
l Improvement method: Optimize welding parameters and reduce the amount of pressure.
Figure 2: Edge burrs
(3)Surface peeling
l Problem description: Surface peeling or threading appears on the surface of the weld in the form of skin or thread.
l Cause: A large amount of heat generated by metal friction accumulates in the surface metal of the weld, causing the local metal on the surface to reach a molten state.
l Improvement method: Optimize welding parameters, reduce rotation speed, and increase welding speed.
Figure 3: Surface peeling
(4) Back weld nodule
l Problem description: Weld bead forms on the back side of the weld.
l Cause: Improper control of the depth and pressure of the stirring head during welding.
l Improvement method: Optimize the depth and pressure settings of the mixing head.
2-Internal defects
(1) Incomplete penetration
l Problem description: A "crack-like" defect occurs when the weld bottom is not connected or is not completely connected.
l Cause: The stirring needle is not long enough, resulting in insufficient stirring of the material at the bottom of the weld.
l Improvement method: Select the appropriate stirring needle length to ensure that the material on the weld thickness can be fully stirred.
Figure 4: Incomplete penetration
(2) Holes
l Problem description: A "crack-like" defect occurs when the weld bottom is not connected or is not completely connected.
l Cause: During the welding process, due to insufficient friction heat input, insufficient material reaches the plasticized state, resulting in insufficient material flow and incomplete closure of the weld area.
l Improvement methods: Optimize welding parameters, improve stirring needle design, and control welding speed and rotation speed.
Figure 5: Holes
We will regularly update you on technologies and information related to thermal design and lightweighting, sharing them for your reference.
Thank you for your attention to Walmate.
Recently, the editor has noticed that many companies are paying attention to and planning immersion liquid cooling energy storage. After a period of silence, immersion liquid cooling technology has attracted attention again and seems to be becoming popular again. I believe that many peers are also paying attention to this development.
1- Features of immersion liquid cooling technology
Energy storage cells are moving towards 300+Ah, and energy storage systems are moving towards 5MWh+. The larger the cell, the more heat it generates, the more difficult it is to dissipate heat, and the more difficult it is to ensure temperature consistency. In addition, the energy storage system is composed of a large number of stacked cells, and the operating conditions are complex and changeable, which is more likely to cause uneven heat generation and uneven temperature distribution. If the problems of heat dissipation and temperature uniformity are not properly solved, the battery's charging and discharging performance, capacity and life will decline, affecting the performance of the entire system. In addition, safety has always been the "Sword of Damocles" hanging over lithium battery energy storage, and the most common way to improve safety is to move towards the three dimensions of intrinsic safety, active safety, and passive safety.
Immersion liquid cooling is to immerse the battery cell in an insulating, non-toxic, heat-dissipating liquid. The coolant has a higher thermal conductivity and specific heat capacity. This direct contact method can provide extremely high heat transfer efficiency while also improving better temperature uniformity. In addition, in addition to being a temperature control medium, the coolant can also be used as a fire-fighting fluid for energy storage systems, combining temperature control and fire protection, which is also a significant feature of immersion liquid cooling technology. Immersion liquid cooling will undoubtedly have more advantages in the context of an industry that requires higher heat dissipation performance and stronger safety.
Figure 1: Immersed liquid-cooled energy storage battery Pack box
2- Immersed liquid cooling energy storage system solution
As a branch of liquid cooling technology, immersion liquid cooling technology is not the first to be used in the energy storage industry. It was initially used in the field of high-performance computing, and later gradually expanded to data centers, artificial intelligence, cryptocurrency, etc.
The original intention of the design of the immersion liquid cooling energy storage system is to solve the shortcomings of traditional air cooling and indirect liquid cooling in cooling efficiency and battery temperature difference control. The official commissioning of the Southern Power Grid Meizhou Baohu project marks the successful application of immersion liquid cooling, a cutting-edge technology, in the field of new energy storage engineering.
l Cooling method and coolant circulation method
The cooling methods are divided into single-phase and phase change. Single-phase immersion liquid cooling is used, mainly including mineral oil, silicone oil, natural ester, etc. Other schemes use two-phase immersion liquid cooling, mainly represented by hydrofluoroether, and use phase change latent heat to dissipate heat and improve heat dissipation efficiency. According to incomplete statistics, the "single-phase immersion cooling" scheme is the most common among the currently released immersion liquid cooling energy storage systems.
According to the difference in the circulation mode of the coolant, there are three technical routes in single-phase immersion liquid cooling: natural convection, pump drive, and immersion coupled cold plate liquid cooling. Natural convection uses the characteristics of liquid volume expansion and density reduction after heating to achieve the floating of hot coolant and sinking after cooling, thereby completing the circulation heat dissipation; the core of the pump drive system is that the liquid cooling unit drives the coolant to circulate between the liquid cooling pipeline and the battery immersion box to complete the entire circulation heat dissipation process; and in the immersion coupled plate liquid cooling scheme, the battery is immersed in the dielectric fluid, and the cold plate in contact with the dielectric fluid is used to take away the heat, avoiding the use of complex secondary circuits to cool the dielectric fluid.
l Product form and integration solution
The integration solution iteration of the immersion liquid-cooled energy storage system is a process from the whole to the part and then to the details. Each step is optimized and improved on the basis of the previous stage to achieve higher performance and safety.
From the cabin level to the pack level, the system integration technology presents the characteristics of scene customization. The diversification of energy storage scenarios makes the demand for energy storage systems different. A single product cannot meet market demand. The modular design allows energy storage products to be optimized and expanded according to the scale and power demand of the project, allowing energy storage solutions to be quickly adjusted and deployed according to different application scenarios and needs.
3-Challenges and implementation scenarios in the industrialization process
Immersed liquid-cooled energy storage systems face many challenges during the commercialization process, including economic feasibility, technical complexity, market acceptance, and industry chain maturity.
l Technical complexity: Compared with cold plate liquid cooling systems, immersion liquid cooling systems are more complex to design and implement.
l Industry chain maturity: The industry chain of immersion liquid cooling technology is not yet fully mature, which limits its application in a wider range of fields. The maturity of the industry chain directly affects the promotion and commercialization of the technology.
l Economic challenges: The energy storage industry is still in the early stages of commercial development, and the lack of profitability makes it difficult for high-cost technology routes to be favored by the market. Many companies compete with low prices for temporary orders, which limits the penetration of immersion liquid cooling.
At present, the main market of the energy storage industry is still dominated by air cooling and cold plate liquid cooling, and immersion liquid cooling has not yet been fully accepted by the market. Although the market penetration and acceptance of immersion liquid cooling technology are not high, it may not show considerable potential in some special scenarios, such as:
l Hazardous chemicals industry: Hazardous chemicals companies have extremely strict safety controls on energy storage equipment, because most of the chemicals they produce and store are highly flammable, explosive, toxic or corrosive. Once an accident occurs, it will not only cause serious losses to the company itself, but may also cause environmental pollution and harm to surrounding communities.
l Base stations and data centers: Base stations and data centers have a low tolerance for thermal runaway. Data center energy storage systems must have batteries with stable performance and are not prone to thermal runaway to ensure system safety. The requirements for power quality are high, and the energy storage system needs to have a fast response capability. In the event of an emergency such as a grid failure or power outage, the energy storage system must be able to directly switch to discharge mode to ensure the continuity and stability of power.
l Fast charging station: When charging and discharging at high rates, the battery generates a large amount of heat in a short period of time, which will cause the battery temperature to be too high and uneven, posing a threat to the battery's performance, life and safety. This means that battery thermal management becomes particularly important in high-rate charging and discharging scenarios.
We will regularly update you on technologies and information related to thermal design and lightweighting, sharing them for your reference.Thank you for your attention to Walmate.
The air tightness of the battery pack is a crucial indicator in electric vehicles and energy storage systems. The air tightness test of the battery pack is mainly carried out on the battery pack shell, interface, connector, cooling assembly, etc. to ensure that the inside of the battery pack is not contaminated or invaded by impurities such as dust and moisture from the external environment, and that the cooling assembly does not leak, so as to ensure that the battery pack maintains normal performance and life, and does not cause safety accidents such as short circuit or explosion.
1-Battery pack protection level and airtightness testing standard formulation
International Protection Making (IEC60529), also known as foreign body protection level or IP Code. The IP (Ingress Protection) protection level system is a standard established by the International Electrotechnical Commission (IEC) to classify the protection level of electrical equipment housings against foreign body intrusion and water intrusion. The airtightness level of the battery pack case is usually required to reach IP67 or IP68, which means that the battery pack case needs to be completely protected from dust ingress (dustproof level 6) and can be immersed in water at a certain pressure for a period of time without water ingress to a harmful level (waterproof level 7). More stringent requirements are that the battery pack can be immersed in 1m deep water for 60 minutes without water ingress (waterproof level 8). The IP protection level usually consists of two digits. The larger the number, the higher the protection level, as shown in Figure 1:
Figure 1: IP protection level description
In order to ensure the battery pack meets the IP67 and IP68 requirements, the battery pack needs to be submerged in water. This method is time-consuming, destructive to the power battery pack, and has certain safety risks. It is not suitable as an offline test for power batteries. Therefore, it has become a common practice in the industry to use airtight testing to ensure that the battery pack meets the IP67 and IP68 requirements. The formulation of airtight testing standards needs to consider the relationship between pressure drop value and leakage rate, as well as the relationship between aperture and water leakage. The formulation of airtight testing standards involves a series of steps from theoretical extremes to experimental verification to achieve the conversion from IP level to airtight testing standards. For example, taking IP68 as an example:
Figure 2: Steps for formulating airtight testing standards
2- Selection of airtightness testing methods and analysis of testing difficulties
The design and manufacturing quality of the battery pack are key factors affecting air tightness, including the toughness and strength of the battery box cover, the sealing of the battery pack shell, interfaces and connectors, explosion-proof vents, and the sealing of the electrical connector itself. In addition, there will be some problems that affect air tightness during use, such as thermal expansion and contraction problems, material aging, and vibration and impact. In the production and manufacturing of battery pack shells, we pay more attention to poor air tightness caused by problems such as welding points and joint quality, such as uneven welding points, weak or cracked welds, air gaps, and poor sealing of joint connections.
The air tightness test of the battery pack is mainly divided into the air tightness test of the upper shell, lower shell, and assembly parts. The air tightness test of the upper and lower shells must meet the air tightness leakage requirements after assembly. When selecting the air tightness test method for the battery pack, the characteristics of the battery pack, test accuracy requirements, production efficiency, and cost are generally considered comprehensively.
Battery pack shell testing in engineering is generally divided into process airtightness testing and shipping airtightness testing. In addition, the airtightness testing of the upper and lower shells must meet the airtightness leakage requirements after assembly, which puts forward more stringent requirements on the testing standards. To ensure that the airtightness meets the requirements, the following difficulties must be overcome in actual operation:
l Product structure stability: the quality of welds, including plug welds, faucet welds, beam welds, frame bottom plate welds, frame front and rear cover plate welds, etc. Weld leakage problems are mainly concentrated in the arc starting and arc ending points and defects caused by burn-through; cracking caused by welding deformation stress, such as bottom plate cavity side wall welding, bottom plate cavity material stratification, and inability to withstand welding deformation stress.
l Adaptability and Stability of Airtight Fixtures: The design of the fixtures should closely match the shape and dimensions of the tested components, ensuring that the components can be securely fixed to the fixtures during the testing process, thereby reducing testing errors caused by positional shifts or vibrations. However, in practice, the size and shape of battery packs vary significantly, necessitating the design and manufacture of multiple different testing fixtures, which increases costs and operational complexity. Designing a universal fixture would further complicate the design process.
l Repeatability of airtightness test results: Factors such as air pressure, temperature, and dryness of the test workpiece/fixture will affect the airtightness test results.
l For workpieces with many non-penetrating tiny cracks, due to the influence of factors such as the accuracy of the detection equipment and the detection parameters, the leakage source may not be discovered, resulting in missed detection.
Figure 3: Airtightness testing tooling
3-Combination of battery pack air tightness detection solutions commonly used in engineering
The air tightness test of the battery pack shell process generally includes air tightness test and water immersion test. In the air tightness test, the upper cover of the battery box is sealed, leaving only a connector port as the air inlet. The air tightness of the battery pack is judged by controlling the air pressure and observing whether there is air leakage. The water immersion test is to completely immerse the entire battery box in water and judge its air tightness by checking whether there is water in the box.
Helium leak detection is a technology that uses helium as a tracer gas to detect leaks by detecting the helium concentration at the leak point. When helium enters the inside or outside of the device being tested where there may be a leak, if there is a leak, the helium will quickly enter or escape the system through the leak and be detected by the mass spectrometer. The helium leak detection method has a high detection efficiency, especially in detecting small leaks.
Figure 4: Comparison of leak detection methods
In actual production, multiple detection methods are usually combined to improve detection efficiency and accuracy. For example, the helium leak detection method is suitable for high-precision and small leak detection, while the differential pressure method has the characteristics of high precision and fast response. In addition, although the traditional water detection method has low detection accuracy, it is intuitive and low-cost, and is a convenient way to locate leaks.
We will regularly update you on technologies and information related to thermal design and lightweighting, sharing them for your reference.Thank you for your attention to Walmate.
The potential failure of the liquid-tightness of the energy storage liquid cooling pack involves multiple aspects, such as: leakage, corrosion and deposition, condensation water and other failure modes.
1- Fluid interconnection and composition
In the energy storage liquid cooling system, the fluid interconnection is responsible for transferring the coolant between the various components. Through effective fluid interconnection, the coolant is ensured to circulate efficiently in the system, thereby removing the excess heat generated during the battery charging and discharging process.
A well-sealed system can effectively prevent coolant leakage. Leakage will not only lead to coolant loss and require frequent replenishment, but also affect the heat dissipation performance and stability of the system. In energy storage, coolant leakage may also cause battery Short circuit, causing safety problems.
2-Liquid-tight design of fluid interconnection system
The liquid-tight design of the fluid interconnection system is the key link to ensure that the system maintains sealing and prevents fluid leakage under various operating conditions.
Figure 1: Typical deployment of energy storage liquid cooling system
(1) Analyze possible leakage sources and risk points in the system:
l The self-sealing property of the liquid cooling assembly. For example, in the integrated design of the liquid cooling channel system and the Pack box, the components are connected by welding. Welding quality defects, poor welding, pores, cracks, etc. may all lead to liquid seepage problems.
l The structural design is unreasonable. For example, the positioning holes or threaded holes of the liquid cooling box are too close to the flow channel, and the poorly welded parts can easily become channels for liquid seepage.
l Connection parts: The pipe connections, valves and joints of the liquid cooling system are common leakage points. If the connection structure is not designed properly or the manufacturing process is not sophisticated, there are tiny defects inside the joints, and the coolant may also leak from these defects.
l Leakage caused by improper installation, material aging or damage, etc.
(2) Sealing structure design:
l The liquid-cooled PACK uses a dry-wet separated cold plate cooling method. Under normal working conditions, the battery cells have no contact with the coolant, which can ensure the normal operation of the battery cells. One solution for the energy storage liquid cooler is to form it through an extrusion process, integrate the flow channel directly on the cold plate, and then use mechanical processing to open up the cooling circulation path. In this process, choosing the right welding process is an important step to ensure sealing. For details, please refer to "Design of welding process for lower box for energy storage".
l Liquid cooling pipelines are mainly used for transitional soft (hard) pipe connections between liquid cooling sources and equipment, between equipment, and between equipment and pipelines. The main connection methods are:
Quick-connection: One of the connection methods for energy storage liquid cooling systems is to use VDA or CQC quick-connection.
Threaded connection: Both ends of the connection structure are slidably connected with pipes, and the threaded connection between the internal thread ring and the threaded sleeve increases the firmness of the connection.
Limiting pipe and nut connection: A connecting pipe is clamped at one end of the pipe, and limiting pipes are fixedly installed on both sides of the connecting pipe. Rubber washers and convex rings are fixedly installed inside the limiting pipes, and a limiting ring groove is opened on the surface of the connecting pipe head. A nut is rotatably connected to the top of the limiting tube and is rotatably connected to the limiting tube through threads.
Sealing ring connection: A sealing ring is adhered to the inner wall of the threaded sleeve by strong glue, and the inner wall of the sealing ring is movably connected to the outer surface of the pipe to prevent leakage during use.
(3) The PACK liquid cooling plate, cabin interface, cabin pipeline, etc. are all designed with long-term corrosion protection under common coolant, common temperature and flow rate conditions to ensure long-term operation without corrosion. Effect of operating conditions on liquid tightness:
l Temperature. Influence of high temperature: As the temperature increases, the viscosity of the liquid generally decreases, which may cause the sealing performance of the liquid to decrease, thereby affecting the liquid tightness. For example, certain sealing materials may deform or deteriorate at high temperatures, causing leakage. Influence of low temperature: In a low temperature environment, the liquid may become viscous, increasing the difficulty of flow, but it may also improve the performance of the sealing material, thereby enhancing liquid tightness to a certain extent.
l Pressure. High pressure environment: Under high pressure, the density and viscosity of the liquid may increase, thereby improving the sealing performance of the liquid. However, excessive pressure may also damage the sealing material and cause leakage. Low-pressure environment: Under low pressure, the sealing performance of the liquid may be relatively weak, especially if the sealing material itself is defective or aged, it is more likely to leak.
l Flow rate. High flow rate: When the liquid flows at high speed, it may produce a large impact force on the sealing surface, causing wear or deformation of the sealing material, thereby affecting the liquid tightness. Low flow rate: At low flow rate, the sealing performance of the liquid is relatively good, but this may also mask some potential sealing problems such as minor material defects.
3-Corrosion and deposition problems
l Effects of blocking on confidentiality:
Coolant, deposits or boiler growth may cause internal blockages, poor coolant flow and reduced cooling efficiency.
Fouling and scaling: Minerals in the coolant may form deposits on the inner wall of the pipe after long-term operation, which is called "scale". Fouling may also be formed due to solid particle precipitation, crystallization, corrosion or microbial activity. These dirt will clog pipes and cold plates, increase flow resistance and reduce heat transfer efficiency.
Foam problem: Foam may be generated in the liquid cooling system. The foam will adhere to the surface of the cold plate, resulting in a decrease in heat transfer effect, and may increase the resistance in system operation, cause cavitation corrosion to the pump, etc., and damage the equipment.
l The influence of eddy current on air tightness:
When a fluid flows in a pipe or gap, changes in velocity can cause eddies to form, especially when the fluid passes through narrow parts or obstacles, eddies are more likely to form. The viscosity and density of the fluid also affect the generation of vortices. Fluids with higher viscosity are more likely to form vortices, while fluids with higher density may weaken the formation of vortices.
Leakage paths: Eddy currents form vortices on contact surfaces, which may form tiny leakage paths in gaps or irregular surfaces, resulting in leakage of gas or liquid.
Surface wear: Eddy flow can cause wear of contact surfaces, especially in high-speed flow conditions. This wear can further reduce air tightness because the worn surfaces are more likely to form new leakage channels.
Thermal effects: Eddy current flow generates heat, which may cause deformation or thermal expansion of the contact surface material, thus affecting the airtightness, especially in systems with large temperature changes.
4-Condensation water problem
Under certain conditions, condensation may form in the liquid cooling lines, which may cause damage to the equipment or reduce efficiency. Insulation failure: If the insulation material of the pipe is damaged or aged, heat will be lost and the cooling effect will be affected. Especially in low temperature environments, insulation failure can cause frost or ice to form on the pipe surface. Frost cracking: In cold environments, if proper antifreeze measures are not taken, the coolant in the pipes may freeze and cause the pipes to rupture.
Solutions
l Sealing measures: Ensure that the inlet and outlet of the liquid cooling pipeline are completely blocked to prevent external humid air from entering the battery compartment.
l Dehumidification equipment: Install a dehumidification air conditioner or use the dehumidification function to maintain the humidity in the battery compartment within an appropriate range.
l Temperature control: By installing air conditioning or ventilation systems, the temperature and humidity of the environment where the energy storage cabinet is located can be controlled. For example, the temperature can be kept at 20-25 degrees Celsius and the relative humidity can be controlled at 40%-60%.
l Isolation measures: Simple isolation of empty battery racks to prevent moisture from entering the compartment containing the battery cluster.
We will regularly update you on technologies and information related to thermal design and lightweighting, sharing them for your reference. Thank you for your attention to Walmate.
The fully sealed design of the energy storage pack is the key to ensuring its safety and long-term stable operation. Sealing is essentially the use of a device to close (seal) a gap or make a joint leak-proof. The fully sealed design can effectively prevent liquid and gas leakage within the battery cell, which is crucial to ensuring the safe and stable operation of the energy storage system. Therefore, when designing, both air tightness and the sealing of the liquid medium must be considered.
In actual operation, the energy storage pack sealing design needs to comprehensively consider multiple factors such as materials, processes, testing equipment, environmental conditions, and manufacturing processes to ensure that its sealing performance can meet the expected standards. This article explains the application practice and key points of energy storage Pack sealing design in actual engineering from the aspects of Pack box airtightness, liquid cooling cycle liquid tightness and liquid cooling medium.
Previous article: Energy storage pack box airtightness design
The sealing design helps maintain the temperature and pressure inside the energy storage pack stable, which plays a key role in the normal operation and performance of the battery; and the sealing design can reduce the impact of the external environment on the internal battery, such as moisture, dust and other pollutants, etc., thereby improving the reliability and service life of the system. In addition, the use of appropriate sealing materials and structures can effectively improve the wear resistance and aging resistance of seals, enhance the durability of the entire energy storage system, and reduce maintenance costs.
The general idea of airtight design is to analyze the box structure to find out the key areas where leakage may exist, and then take targeted measures according to the specific performance and functional requirements of different areas.
1-Box structure analysis
The box is not only the physical carrier of battery modules and electrical components, but also an important guarantee for the safe and reliable operation of the entire energy storage system. It is the "skeleton" of the energy storage pack, which is generally composed of an upper cover, a lower box, support components, and a sealing Parts and bolts, etc.
Figure 1: Schematic diagram of the energy storage pack box and key areas of focus in the sealing design (e.g., marked with red arrows)
As shown in the figure above, find out where potential leaks may occur:
l Multiple parts connection points, such as: the assembly interface between the upper cover and the lower box, the installation interface between the high and low voltage connectors and the box, the installation interface between the exposed components and the battery box, etc.
l If bolts are used for connection, there may also be a risk of leakage at the installation and fixing point, such as the electrical interface and the front panel installation interface of the box.
l There must be no holes or gaps in the upper cover and lower body of the box to ensure the sealing and protective performance of the box.
Figure 2: Immersion liquid cooling lower box (sheet metal frame + aluminum liquid cooling bottom plate)
2- Sealing design of the installation interface between the upper cover and the lower box
The upper cover can generally be divided into two types: flat type and special-shaped type. Their structural characteristics are also different. For example, SMC composite material, aluminum, no matter what material, in order to reduce the complexity of the self-sealing structure, the upper cover of the battery shell The cover is usually of one-piece design. In addition, the opening requirements of the upper cover should also meet the requirements of the interface, and should be independent of the sealing interface to reduce the impact on the battery pack sealing. The upper cover seal design generally follows the following principles:
l The integrated parts design is adopted to avoid the design of separate parts, thus ensuring the stability of the "self-sealing" performance of the upper cover.
l The positioning holes and positioning features are designed on the edge of the upper cover (outside the sealing interface between the upper cover and the lower tray).
l The sealing interface between the upper cover and the lower box body requires the matching surface to meet the "uniform" and "continuous" sealing requirements.
At present, the mainstream solutions for the lower box of the energy storage pack are: sheet metal box + liquid cooling plate, die-cast box + liquid cooling plate, profile integrated box, die-cast integrated box, etc. Among them, the profile integrated box and other solutions In contrast, it has the advantages of good flow channel bearing capacity and low mold opening cost, and is widely used. The choice of welding process has a great influence on the sealing performance. For weldments of different materials and thicknesses, choosing a suitable welding method can effectively improve the weld quality to ensure the overall strength and sealing performance of the system.
In addition, the sealing design of the lower box should follow the following sealing principles:
l Closed section profiles are used for frame construction, and self-sealing linear connection technology, such as CMT welding technology, is used at the joints.
l Battery trays made of aluminum profiles need to be designed with one or more continuous layers of sealing colloid.
l In the case of an integrated liquid cooling plate in the lower box, it is necessary to consider using colloid seals or self-sealing linear connection technologies, such as FSW friction stir welding technology.
l The sealing interface between the upper cover and the lower box body needs the matching surface to meet the "uniform" and "continuous" sealing requirements. If necessary, the sealing interface should be machined and polished.
Figure 3: Common sealing forms between the upper cover and the lower box
Usually, the upper cover and lower box of the energy storage Pack box adopt a bent flange and a sealing gasket design, as shown in Figure 2. The upper cover, lower box body and sealing gasket are fully compacted and coupled by fastening bolts to ensure that the Pack box meets the relevant requirements of IP67.
3- Sealing design of electrical and communication interfaces and the front panel installation interface of the lower box
The front panel of the box (as shown in Figure 3) is machined with holes on the extruded profile for installing electrical and communication interfaces to achieve functions such as current transmission, communication interaction, and safety control.
Figure 4: Electrical, communication interface and lower cabinet front panel installation interface
The air tightness of the installation interface between the box and the electrical, communication and other interfaces shall follow the following principles:
l The interface shape is designed to be streamlined to reduce the possibility of gas and liquid accumulation and penetration at the interface.
l Precise alignment avoids gaps caused by misalignment of interfaces during installation.
l Pre-seal the interface before installation and add anti-vibration pads or sealants to enhance the initial sealing effect or reduce sealing failure caused by vibration.
In addition, in terms of the selection of fasteners, high-strength, high-torque fasteners are used, and they are tightened multiple times during the installation process to ensure the tightness of the interface. For example, if a butt-weld nut is used, its characteristic is that it can be directly connected to the wall hole of the connected part (the front panel of the box) for butt welding. This structural design can significantly improve the airtightness of the connection part.
Figure 5: Using butt-welded nuts to increase airtightness
4-Seal selection
Seal design and selection are critical as they directly affect the reliability and service life of the system. The following are key factors to consider when designing and selecting seals for energy storage liquid cooling systems:
l The sealing material must have certain chemical and pressure compatibility, and be able to withstand the system operating temperature range, including high and low temperature environments. The material selection of the seal depends on the use environment and service life requirements. Common sealing materials include rubber, polytetrafluoroethylene (PTFE), nylon, metal, etc.
l Leakage freedom: The seal must be able to adapt to the slight deformation that may occur in the system during operation to ensure good sealing effect under various working conditions. Generally, the deformation of the gasket should be greater than 30% and less than 60%, and the sealing interface pressure should be greater than 30kPa.
We will regularly update you on technologies and information related to thermal design and lightweighting, sharing them for your reference.Thank you for your attention to Walmate.
In pure electric vehicles, the weight of the battery pack accounts for about 30% of the curb weight. The lightweight of the battery pack is of great significance to improving the cruising range of the vehicle. Therefore, research on the high specific energy of battery packs is one of the current main research directions for new energy vehicles, and it is also the main way to achieve lightweight electric vehicles. The lightweighting of power battery packs can be carried out in two directions: improving single cells The energy density of the battery pack is optimized, and the related accessories of the battery pack are optimized.
The development of multi-material lightweight battery packs aims to reduce the weight of the battery pack, increase energy density and cruising range, while ensuring safety and reliability by using a variety of lightweight materials. Among the main components of the battery pack, the battery cell body has the highest mass, followed by the lower box of the pack, the upper cover, and BMS integrated components.
1-Lightweight design of the battery pack cover
The upper cover of the battery box is located above the power battery box and is not affected by the sides of the power battery box and will not affect the quality of the entire battery pack. Its functions mainly include sealing and protection. In order to improve the energy efficiency of the entire vehicle, lightweight materials will also be considered in the design. The use of lightweight materials, such as aluminum alloys and composite materials (SMC, FRP, etc.), can significantly reduce the weight of the battery pack.
In addition, the structural design of the upper cover also needs to consider manufacturing efficiency and mass production requirements. When the structure is very irregular, it may be difficult to use stamping forming or bending and tailor welding. The design of the upper cover also needs to consider the connection and cooperation with other components such as the lower box and sealing structural parts to ensure the structural stability and reliability of the entire battery box.
2-Lightweight design of the battery pack lower shell
Aluminum alloy is an ideal material for battery pack shells because of its low density, high specific strength, good thermal stability, strong corrosion resistance, good thermal conductivity, non-magnetic, easy molding and high recycling value. Commonly used aluminum alloy materials include 6061-T6, 6005A-T6 and 6063-T6. These materials have different yield strengths and tensile strengths and can meet different structural needs.
The battery pack shell is usually composed of an aluminum alloy profile frame and a bottom plate, which is welded using 6 series aluminum alloy extruded profiles. Factors such as the size and complexity of the profile section, wall thickness, etc. need to be considered during design to adapt to different structural and functional requirements. For example, components such as frames, middle partitions, floor panels, beams, etc. may adopt different cross-section designs.
Through reasonable structural design and connection methods, the overall strength of the shell can be effectively ensured, the processing difficulty can be reduced, and the weight of the shell can be reduced:
l Thin-walled: By adopting a thin-walled design and using stiffeners to meet strength requirements, the weight of the material can be effectively reduced.
l Hollowing: Introducing hollow sections into structural design to reduce material density.
l Size optimization: Optimize the size of the battery pack to reduce unnecessary material usage.
l Topology optimization: Reduce the space occupied by materials by optimizing the layout of internal components of the battery pack.
l Integrated modular design: Integrate cooling plates, battery pack lifting lugs and other components into the box to reduce the number and weight of individual components.
3-Manufacturing lightweight technology
l Material shaping
At present, there are three main categories of battery box material forming processes: stamping, aluminum alloy die-casting and aluminum alloy extrusion. The overall process flow of the power battery box includes material molding and connection processes, among which the material molding process is the key process of the power battery box. At present, the upper casing is mainly stamped, and the main processes of the lower casing are extrusion molding and aluminum alloy die-casting.
l Connection technology
The battery box connection process is crucial in new energy vehicle manufacturing and involves a variety of technologies and methods to ensure the structural strength and sealing of the battery box.
Welding is the main connection process in battery box processing and is widely used. Mainly include the following methods:
① Traditional fusion welding: such as TIG (tungsten inert gas welding) and MIG (metal inert gas welding). TIG welding has low speed and high quality. It is suitable for spot welding and complex trajectory welding. It is often used for frame tailor welding and side beam small piece welding. MIG welding has high speed and strong penetration ability, and is suitable for full-circle welding inside the frame bottom plate assembly.
② Friction stir welding: Welding is achieved by generating heat through friction. It has the characteristics of good joint quality and high production efficiency.
③Cold metal transfer technology: It is suitable for thin plate materials. There is no heat input during the welding process, reducing deformation.
④Laser welding: high precision, high speed, suitable for complex structure welding.
⑤ Stud welding and projection welding: used for quick connection of specific parts. Stud welding is fastened by studs and nuts, and projection welding is connected by pressing bumps.
The mechanical connection method mainly solves the problems of easy welding and thread slippage of thin plate materials during welding, including:
① Blind rivet nut: used to connect the sealing surface of the box frame and the inner cavity bottom plate. It has the advantages of high fastening efficiency and low use cost. Suitable for threaded connections between thin plates and other components.
②Wire thread insert: used to strengthen the screw holes of aluminum or other low-strength bodies, improve the load-bearing capacity of the screws and the force distribution of the threads, and is suitable for battery module mounting holes and sealing surface mounting holes. Compared with blind rivet nuts, wire thread inserts are stronger and easier to repair, but are generally not suitable for thin-wall installations.
We will regularly update you on technologies and information related to thermal design and lightweighting, sharing them for your reference.
Thank you for your attention to Walmate.
In order to cope with market demands such as large spans, fast iterations, and rich product lines, while ensuring cost reduction, efficiency improvement, and quality assurance, for the automotive industry, product standardization - vehicle platformization is undoubtedly a good strategy. Through battery platformization, the same battery pack solution can be matched for different models, or battery pack solutions composed of the same type of battery cells and similar structures can be matched. This means that as many parts as possible can be standardized, which can shorten the development cycle, save costs, streamline production lines, and improve production efficiency.
ONE:Battery Platformization
The battery platform solution is conducive to the overall planning of products, cost reduction and optimization of production capacity. According to the battery platform strategy of the vehicle platform, it is necessary to consider the intersection and bandwidth of the requirements of each model of the platform, and use as few batteries and battery solutions as possible to be compatible with as many models as possible. In the architecture development of pure electric projects, it is crucial to reasonably arrange the integrated power battery pack. Specific work elements include power and power performance requirements, collision safety, layout location and space, etc.
1-Spatial size boundaries and battery cell standardization
l Available battery pack locations
At present, the mainstream power battery layout is under the floor, including under the front seats, under the rear seats, in the middle channel and at the footrest. This layout can maximize the available area, help lower the center of gravity of the vehicle, improve the handling stability of the vehicle and optimize the collision force transmission path.
Figure 1: Battery pack layout during the development of electric vehicles
l Evolution of battery pack space layout
Split battery pack: A split battery pack space layout is adopted, such as the JAC Tongyue series. The energy module consists of two battery packs, one placed at the original fuel tank position, and the other placed in the trunk where the spare tire is stored.
In addition, engineers are continuously exploring usable space within the original architecture of fuel vehicles, resulting in battery pack layouts appearing in "工" (gong), "T," and "土" (tu) shapes.
This type of design is a minor modification of a traditional fuel vehicle. The space is very limited, and the volume and weight of the battery pack that can be loaded are very limited, so the capacity is difficult to increase and the cruising range is not high.
Integrated battery pack: This is a new product design concept. The design of the entire vehicle revolves around the core component - the battery pack. The battery pack is modularly designed and laid flat on the vehicle chassis to maximize available space.
l Battery pack installation point layout
Reasonable layout of the battery pack is crucial, and the limiting factors in the design are ground clearance, passability, collision safety, power requirements and many other aspects.
Figure 2: Battery pack size design constraints
The vehicle platform needs to define the category, level and positioning of each vehicle model within the platform, and then determine the size and wheelbase of the vehicle. The vehicle layout decomposes the size envelope of the battery pack in the X, Y and Z directions according to the vehicle space. The battery needs to be arranged within the given envelope of the vehicle to ensure that there is no interference between the various systems of the vehicle. The curb weight index can decompose the system quality requirements of the battery pack.
In terms of battery size, the design of power battery packs cannot avoid rigid reference indicators such as vehicle space and curb weight, which means that there is a threshold for the design of battery cells. Constrained by this threshold, the battery cell size will be concentrated in a certain range, such as: the length of square battery cells ranges from 150-220mm, the width ranges from 20-80mm, and the height ranges around 100mm. The changing trend of battery cell size specifications is the result of the complementary relationship between vehicle platformization and battery standardization.
However, the battery platform strategies, vehicle models and understanding of standardization of various automakers are different, resulting in significant differences in the current product solutions. For example, BYD's standardization strategy is to fully replace the blade battery, the size of which is locked at 960*13.5 (14)*90 (102) mm, and the single cell voltage is 3.2/3.3V.
2- Development of Endurance Boundaries and Battery Capacity Solutions2- Development of Endurance Boundaries and Battery Capacity Solutions
The power battery provides energy for the vehicle to travel: battery capacity, discharge depth, and energy density affect the amount of power available. In order to meet the needs of different models, the difference in power consumption of models has become an important concern. The vehicle's cruising range will be affected by factors such as electric drive, battery, curb weight, wind resistance, mechanical resistance, low-voltage power consumption, and energy recovery. The possibility of sharing battery solutions between models with large differences in power consumption is weak, so it is necessary to develop personalized battery power solutions, including battery size, quality, power, and power performance optimization to meet the requirements of cruising performance.
Under the constraints of the pure electric range of the vehicle manufacturing platform, the net discharge required by the battery will be affected by the power consumption of different models. It is necessary to confirm the power consumption distribution of each model on the platform in order to further convert the power consumption bandwidth into the battery demand distribution, and then determine the battery power plan required by the platform.
3-Power performance boundary
The dynamic performance of the whole vehicle includes the power performance under acceleration conditions, constant speed conditions and power preservation conditions at different SOCs and ambient temperatures. The variables corresponding to the battery are the power-voltage characteristics of the battery at different SOCs and temperatures. The power of the battery corresponds to the power requirement of the vehicle's power system, and the voltage corresponds to the rated voltage requirement of the drive motor.
Generally, the evaluation of battery solutions for the entire vehicle platform starts from the acceleration time of 100 kilometers at normal temperature and high power and its battery indicator decomposition, and gradually extends to the battery indicator decomposition over the entire range and under all operating conditions.
SECOND:Battery box development
1-Battery integration and modularization
Optimize the design of battery modules, improve the integration and modularity of battery packs, reduce inactive components, and increase the energy density of battery packs.
Currently popular battery pack integration technologies include CTP, CTB, CTC and other forms. The shape, material and combination of parts have changed with the advancement of integration technology. The overall direction is integration and integration. By reducing the number of independent parts and using one large part to replace several parts, larger and more functional components are formed.
2-Battery box design
The battery case is the carrier of the power battery system assembly, plays a key role in the safe operation and protection of the product, and directly affects the safety of the entire vehicle. The structural design of the battery case mainly includes the selection of shell materials for the upper shell, lower shell and other components of the battery case, and the selection of manufacturing process solutions. The upper cover of the battery case mainly plays a sealing role and is not subject to much force; the lower case of the battery case is the carrier of the entire power battery system product, and the battery module is mainly arranged in the lower case. Therefore, there must be structural measures such as embedded grooves and baffles inside the battery case to ensure that the battery module is reliably fixed when the vehicle is driving, and there is no movement in the front, back, left, right, up and down directions, so as to avoid impact on the side walls and upper cover and affect the life of the battery case.
Figure 3: Battery lower box solution, a-skin frame, b-FSW welding + frame, c-FSW welding + frame
l Battery pack installation point structure design and connection fixation
The battery pack installation point usually adopts a mounting beam structure, which runs through the front and back, and the front end is connected to the front cabin longitudinal beam to form an effective and coherent closed beam structure. The installation points are reasonably arranged according to the weight distribution of the battery pack. The battery pack and the vehicle are fixed in various ways, including bolt fixing, mechanical fixing + adhesive joint hybrid connection, snap-on connection, etc.
Figure 4: Battery pack layout and installation section
The power battery pack is generally installed on the vehicle through multiple lifting lug structures. In addition to the large weight of the power battery pack itself, the lifting lugs must also withstand the road excitation brought by the vehicle's movement, such as stone roads and deep potholes. Such durable working conditions and misuse conditions place higher requirements on the strength of the lifting lug structure.
Figure 5: Different lifting lug connection solutions: a Welded lifting lug b Aluminum extruded frame lifting lug
l Battery box safety and protection structure
Mechanical strength and protection: The battery box should have sufficient mechanical strength to protect the batteries inside from mechanical shock and impact. The battery box needs to be able to withstand vibration, extrusion, and mechanical shock to ensure the safety of the battery under various conditions.
Collision protection: The design of the battery case must take collision safety into consideration, especially for side collisions and bottom collisions. It is usually made of aluminum or steel and connected to the lower tray through an outer frame to provide structural rigidity and enhance collision energy absorption capabilities. In addition, appropriate collision absorption structures should be designed to prevent deformation of the battery case and damage to the battery cells.
Waterproof, dustproof and corrosion-resistant: The battery box needs to be waterproof and dustproof, and usually uses IP67-level sealing gaskets to ensure airtightness. In addition, anti-corrosion measures should also be considered, such as spraying PVC coating on the outside to enhance corrosion resistance.
Explosion-proof and pressure relief design: When a battery explodes, the energy should be released in a concentrated and directional manner through devices such as balanced explosion-proof valves to avoid entering the customer cabin. In addition, explosion-proof measures (such as partial structural destruction) should be taken to prevent the overall rupture of the equipment.
l Sealing design
The design of the sealing surface between the upper cover and the lower case of the battery box plays an important role in the sealing performance, and its design needs to be designed together with the battery box structure and the sealing ring. The sealing surface should be kept in the same plane as much as possible to avoid too many curved structures. Since the upper cover and the lower case are connected by bolts, a large number of bolts are used, so it is particularly important to ensure the coaxiality of the holes. While arranging the bolt hole positions reasonably, the position dimensions should be as round as possible and arranged symmetrically in the X and Y directions. The selection of the number of connecting bolts needs to be comprehensively considered based on the level of sealing and the amount of disassembly and assembly workload.
Figure 6: Upper and lower box sealing design, 1-battery upper cover 2-sealing gasket 3-battery lower cover 4-metal conduit
l Electrical safety and short circuit protection
Connection reliability: The connectors inside the battery box should have the correct polarity connection to ensure the overcurrent capacity of the battery box and the reliability of the electrical/mechanical connections, including relaxation measures, etc.
Electrical insulation and voltage resistance design: The module design adopts double insulation protection. The battery cell itself has a layer of battery cell blue film and a battery cell top patch to meet the insulation and voltage resistance requirements. Insulation and voltage resistance protection is set between the end/side plate and the battery cell, and between the battery cell and the bottom mounting surface.
l Thermal management design
Battery thermal management development runs through the entire cycle of battery pack system design and development, including the design of battery temperature control, cold plate, piping system, etc. The main goal of battery thermal management system design is to ensure that the battery system operates at a relatively suitable operating temperature through heating or cooling control while considering space layout, design cost, lightweight, etc., while reducing the temperature difference between cells to ensure consistency.
We will regularly update you on technologies and information related to thermal design and lightweighting, sharing them for your reference.Thank you for your attention to Walmate.
The battery pack is the core energy source of new energy vehicles, providing driving power for the entire vehicle. We generally evaluate the advantages and disadvantages of battery pack technology from the dimensions of efficiency (energy density), safety, manufacturing and maintenance costs.
In battery design, the voltage of a single cell is only about 3-4V, while the voltage required by electric vehicles is at least 100V. New cars now even have a voltage of 700V/800V, and the output power is generally 200W, so the battery needs to be boosted. In order to meet the current and voltage requirements of electric vehicles, different cells need to be connected in series or parallel.
The battery pack is composed of battery cells, electronic and electrical systems, thermal management systems, etc., which are enclosed by a battery frame structure - base plate (tray), frame (metal frame), upper cover plate, bolts, etc. How to "package" these components and systems into a whole more efficiently and safely has always been a topic of continuous research and exploration for the entire industry.
Previous article: Innovation and development of battery integration technology
The origin of power battery group technology can be traced back to the 1950s, and originated in the former Soviet Union and some European countries. This technology was originally used as an engineering and manufacturing concept to determine the physical similarities of parts (universal process routes) and establish their efficient production.
The core of Group Technology (GT) is to identify and explore the similarities of related things in production activities, classify similar problems into groups, and seek relatively unified optimal solutions to solve this group of problems to achieve economic benefits. In the field of power batteries, group technology mainly involves the technology of integrating batteries from single cells into battery packs (Packs), including structure, thermal management, electrical connection design and battery management system (BMS) technology.
The earlier grouping technology in the automotive field is MTP (Module To Pack), which means that the cells are first integrated into modules, and then the modules are integrated into Packs. This technology is characterized by detachable and replaceable modules, which have good maintainability, but the grouping efficiency is low. With the development of technology, the grouping technology has undergone a transformation from MTP to CTP (Cell To Pack). CTP technology refers to the technology of directly integrating cells into Packs, eliminating the traditional module structure and improving grouping efficiency and production efficiency. In recent years, the industry is also exploring grouping technologies such as CTC (Cell To Chassis), CTB (Cell To Body & Bracket) and MTB (Module To Body) with higher integration efficiency.
In the field of power batteries and electrochemical energy storage, the main technological advances of lithium batteries come from structural innovation and material innovation. The former is to optimize the structure of "cell-module-battery pack" at the physical level to achieve the goal of both improving the volume energy density of the battery pack and reducing costs; the latter is to explore battery materials at the chemical level to achieve the goal of both improving the performance of single cells and reducing costs. This article focuses on the impact of different structural integration technologies on battery pack manufacturing technology and the direction of innovative development from the perspective of battery pack structural integration. The current key technologies for power battery integration are shown in the figure below:
1-MTP has been eliminated
At the beginning of the current wave of electric vehicle development, many oil-to-electric new energy vehicle models have been launched. They continue the spatial layout and styling design of traditional gasoline vehicles. Engineers have assembled a relatively large battery cell module by connecting a certain number of individual battery cells in series/parallel, and then placed several such battery cell modules into the battery pack, which is the familiar "MTP" battery pack. Since the battery pack needs to be "packaged" more than twice, the number of required components is extremely large, and the battery pack appears as "three layers inside and three layers outside", with too many redundant parts occupying more system volume and weight, resulting in poor volumetric energy density and gravimetric energy density of the "MTP" battery pack. Additionally, since the design of gasoline vehicles did not specifically reserve space for the battery, the battery system can only be "squeezed in wherever it fits", leading to poor product competitiveness and user experience.
Since the launch of new intelligent electric vehicle platforms represented by Tesla, native pure electric vehicles have enabled battery packs to be installed in ideal spatial locations in a more efficient and regular manner, the three-electric systems can be more reasonably laid out, and the vehicle's electronic and electrical architecture and thermal management design can be more efficiently integrated. The vehicle's product strength in terms of energy efficiency, endurance, and intelligence has been greatly enhanced.
2-Integrated Technology 2.0 Era——CTP
The MTP structure battery pack has a significant space utilization problem. The space utilization of the battery cell to the module is 80%, the space utilization of the module to the battery pack is 50%, and the overall space utilization is only 40%. The module hardware cost accounts for about 14% of the total battery cost. This low space utilization structure cannot meet the development requirements of new energy vehicles. Under the framework of the battery cell → module → battery pack → body integration idea, if the vehicle wants to load as much power as possible in the limited chassis space and improve the volume utilization, it is necessary to consider the standardization of each integration step. As the market demand for driving range continues to rise, the volume of a single battery module continues to increase, which indirectly leads to the emergence of the CTP solution.
CTP structure technology was born for the consideration of safety, packaging complexity, cost reduction, etc. Under the premise of ensuring the safety of the battery cell, CTP technology reduces the internal cables and structural parts. Compared with MTP technology, CTP technology has no module structure and directly packages the battery cell into a battery pack before installing it on the vehicle.
There are currently two main ideas. One is to regard the Pack as a complete large module that replaces the structure of multiple small modules inside, represented by CATL; the other is to consider using a module-free solution during design, and design the battery itself as the strength participant, such as BYD's blade battery.
The core point of CTP technology is to cancel the module design. The battery cell is directly combined with the shell, reducing the use of end plates and partitions. The problems that follow are the fixation of the battery pack and thermal management.
In fact, the original product of the CTP battery pack was not a pure module-free design, but a design that merged the original small modules into three large modules and two medium modules, and there were also aluminum end plates at both ends, so in theory it is still MTP, but there are indeed great improvements in structure.
After the introduction of CTP 3.0, CATL presented a more advanced manufacturing method, achieving a completely module-free design. The battery cells have been changed from a vertical orientation along the height to a horizontal position. Additionally, a new cooling solution has been implemented between the battery cells, which not only dissipates heat but also provides support, cushioning, insulation, and temperature control functions. The bottom shell has also been designed with a limiting fixation feature.
Figure 1: Comparison between CATL Kirin Battery CTP2.0 and CTP3.0
3-Integrated Technology 3.0 Era——CTB, CTC
a.CTB technology
CTP technology is a major step forward in battery structure innovation, but it has not made a breakthrough in the battery pack itself. In CTP technology, the battery pack is still an independent component. Compared with CTP's streamlined strategy for battery packs, CTB technology combines the body floor panel and the battery pack cover into one. The flat sealing surface formed by the battery cover, the door sill, and the front and rear beams seals the passenger compartment with sealant, and the bottom is assembled with the body through the installation point. When designing and manufacturing the battery pack, the battery system is integrated with the body as a whole, the sealing and waterproof requirements of the battery itself can be met, and the sealing of the battery and the passenger compartment is relatively simple, and the risks are controllable.
In this way, the original sandwich structure of "battery pack cover-battery cell-tray" is transformed into a sandwich structure of "body underbody integrated battery pack cover-battery cell-tray", reducing the space loss caused by the connection between the body and the battery cover. In this structural mode, the battery pack is not only an energy source, but also participates in the force and transmission of the whole vehicle as a structure.
Figure 2: Schematic diagram of CTB technology structure
b.CTC technology
After adopting the CTC method, the battery pack is no longer an independent assembly, but is integrated into the body of the vehicle, which optimizes the product design and production process, reduces the number of vehicle parts, especially reduces the internal structural parts and connectors of the battery, has the inherent advantage of lightweight, maximizes the space utilization, and provides space for increasing the number of batteries and improving the driving range. Under the condition that the electrochemical system itself remains unchanged, the driving range can be increased by increasing the number of batteries.
Figure 3: Tesla CTC technology structure diagram
For example, Tesla and other automakers have successively launched CTC technology models. At the cell level, they can use multifunctional elastic sandwich structures and large-area water cooling technology, and superimpose the anti-collision space reuse technology at the bottom of the battery pack brought by integrated development, taking into account the grouping efficiency, heat dissipation and safety, and promoting the application of CTC technology from the two dimensions of cell optimization and vehicle structure protection. At the level of vehicle integrated development, the battery cell is directly integrated into the chassis, eliminating the links of modules and battery packs. The integration of the three major electric systems (motor, electronic control, battery), the three minor electric systems (DC/DC, OBC, PDU), the chassis system (transmission system, driving system, steering system, braking system) and autonomous driving related modules is realized, and the power distribution is optimized and energy consumption is reduced through the intelligent power domain controller.
4-Changes in specific requirements for battery boxes for CTP, CTB and CTC technologies
In the traditional battery pack structure, the battery module plays the role of supporting, fixing and protecting the battery cell, while the battery box body mainly bears the external extrusion force. The application of CTP, CTB and CTC technologies puts forward new requirements for battery boxes, which are specifically reflected in:
The strength requirements of the battery box body are improved: Since the module link is reduced or eliminated in the CTP, CTB and CTC structures, the battery box body must not only withstand the external extrusion force, but also the expansion force from the battery cell originally borne by the module. Therefore, the strength requirements of the battery box body are higher.
Collision protection capability: After using CTP technology to remove the side beams of the battery pack, the battery will directly bear the impact of the collision, so the CTP battery pack needs to have sufficient collision resistance.
Insulation, insulation and heat dissipation requirements: CTP or CTB and CTC structures change the bottom plate profile to a water-cooled plate based on the chassis-bearing structural box. The battery box box not only bears the weight of the battery cells, but also provides thermal management and other functions for the battery. The structure is more compact, the manufacturing process is optimized, and the degree of automation is higher.
Reduced maintainability: The highly integrated design makes it complicated to replace the battery pack. For example, in the CTC structure, the battery cells are filled with resin material, which makes it difficult to replace the battery cells and almost impossible to repair.
5- Impact of battery pack integration on electric vehicle charging infrastructure
Choosing different battery pack integration technologies also implies choosing different compensation methods. CTP tends to be battery replacement, while the more highly integrated CTB/CTC tends to be fast charging.
High integration means that more batteries can be accommodated in the same space, thereby increasing the range of electric vehicles. Users may no longer need to charge frequently for short distances, but may prefer to charge quickly during long journeys. Therefore, the planning of charging infrastructure needs to take these changes into account to ensure that it can meet user needs.
As the integration of battery packs increases, the physical size and structure of the battery packs may change, which may affect the design of the charging interface and the compatibility of the charging equipment.
In addition, the increased integration of battery packs may also affect charging speed and efficiency. More efficient battery management systems and charging technologies may need to be developed and deployed to ensure a fast and safe charging process.
We will regularly update you on technologies and information related to thermal design and lightweighting, sharing them for your reference.
Thank you for your attention to Walmate.
In the production process of battery trays and energy storage liquid cold boxes for new energy vehicles, necessary and appropriate surface treatment is a key step, such as: using coating, oxidation treatment, etc. to form a protective layer on the metal surface to resist the erosion of corrosive media; Components that require electrical isolation, such as battery cells, water-cooling plates, module walls, etc., need to establish an insulating protective film. Insulation is generally achieved by spraying insulating powder or insulating paint. Choosing the appropriate surface treatment technology can not only improve the performance of the tray/liquid cooling box Durability and safety can also meet the needs of different application scenarios. This article summarizes common surface treatment technologies for reference.
1-Cleaning and polishing
During the production process, impurities such as processing oil, engine oil residue, powder, and dust may accumulate on the surface of the pallet. Not only do these impurities affect the service life of the battery tray, they may also adversely affect the performance and safety of the battery. Through cleaning and polishing, these impurities can be effectively removed to ensure the cleanliness of the pallet surface. Cleaning and grinding can effectively remove surface impurities, burrs, and welding slag, making the surface smooth and flat, thus improving the overall quality of the battery tray/box.
a.chemical cleaning
Alkali cleaning: Alkaline cleaning mainly uses alkaline solutions (such as sodium hydroxide, sodium carbonate, etc.) to remove grease, dirt and other organic matter on the surface of aluminum alloys. Alkaline washing removes grease through saponification, emulsification and penetration and wetting, and at the same time generates water-soluble precipitates, thereby achieving a cleaning effect. Alkaline cleaning is usually used to remove grease, dust and organic contaminants from the surface of aluminum alloys.
Pickling: Pickling uses acidic solutions (such as nitric acid, hydrochloric acid, etc.) to remove oxide scale, rust and other inorganic deposits on the surface of aluminum alloys. Pickling converts the oxides on the metal surface into soluble salts through the reaction of acid with the oxides on the metal surface, thereby removing surface impurities. Pickling is mainly used to remove oxide film, rust and inorganic salt scale on the surface of aluminum alloys. Pickling is often used for the final treatment of metal surfaces to improve their finish and flatness.
b.Mechanical grinding
During production, the grinding process can remove processing allowances, correct shape errors, ensure the smoothness and accuracy of the pallet/box surface, meet assembly requirements, and thus improve overall performance and service life.
The cleaned and polished surface can be treated with coating materials or other materials, which is very important for the subsequent construction of anti-corrosion, sealing, thermal conductivity, insulation, thermal insulation and other coatings, and plays a key role in the firm attachment of these materials to the pallet/box.
2-Coating and protective film establishment
In addition to basic cleaning and polishing, the production of pallets/boxes uses a spraying process for surface treatment to form a protective layer to prevent oxidation and corrosion and to meet the needs of different scenarios such as thermal insulation, insulation and voltage resistance.
a.Thermal insulation
Anti-condensation and thermal insulation of battery trays can be achieved through comprehensive design of thermal insulation systems, use of high-efficiency thermal insulation materials, application of aerogels, battery pack insulation design, and spraying of foam insulation materials.
Bottom surface sprayed with PVC and foam material
b.Insulation withstand voltage
The insulation of the battery pack casing and the liquid cooling components is primarily to prevent current leakage, protect personnel from electric shocks, and ensure the normal operation of the battery system. Insulation is typically achieved through two main methods: powder spraying and film lamination. The mainstream film lamination processes include room temperature lamination, hot pressing, and UV exposure.
Internal spraying of insulation powder and insulation paint
3-Logos and Signage
A nameplate or label is set in a prominent position on the battery tray, generally through laser, mechanical engraving, etc. These logos are usually made of wear-resistant and corrosion-resistant media to ensure that they are not easily erased during the entire service life.
We will regularly update you on technologies and information related to thermal design and lightweighting, sharing them for your reference.
Thank you for your attention to Walmate.
As the core equipment of the energy storage system, the energy storage converter is an important tool for power conversion, energy management, ensuring grid stability, improving energy efficiency, etc. As the energy storage converter power unit moves toward high integration and high efficiency, the development of frequency and large capacity puts higher and higher requirements on heat dissipation.
1-Changes in cooling requirements
a.Matching the larger DC cabin, the converter capacity continues to increase, and efficient heat dissipation technology ensures the reliability of the equipment.
As the capacity of energy storage cells becomes larger and larger, the capacity of energy storage systems is also expanding simultaneously. At the beginning of 2023, the standard 20-foot single-cell battery capacity on the market was only 3.35MWh. In the second half of the year, many battery cell companies have launched 310+Ah energy storage products, and the capacity of the 20-foot single-cell battery has also been expanded to 5MWh. However, less than half a year after the 5MWh model was updated, some leading energy storage systems released 6MWh and 8MWh systems. According to general experience, the energy storage converter is configured at 1.2 times the load capacity. The single unit capacity of a 5MWh energy storage system must be greater than 2.5MW. High power requires more efficient cooling technology to ensure stable operation of the equipment under sustained high loads.
Iterative evolution of energy storage system integration topology scheme
b.The application of DC high-voltage technology requires devices to have higher withstand voltage levels and insulation strength, and the heat dissipation of power devices is severe.
In order to match the large-capacity energy storage system, DC high-voltage technology has become a technical trend. Through the increase of voltage level, energy saving, efficiency and performance improvement can be achieved. The 1500V voltage upgrade originated from photovoltaics, and now photovoltaics are involved in energy storage. However, the high-voltage evolution of energy storage PCS still has a long way to go, and some manufacturers have begun to optimize and push it to 2000V. The application of DC high-voltage technology forces the power electronic devices in energy storage converters to have higher withstand voltage levels and higher insulation strength to adapt to high-voltage working environments. In high-voltage environments, the heat dissipation design of power devices becomes more important. The pn junction temperature of power devices generally cannot exceed 125°C, and the temperature of the package shell does not exceed 85°C.
c.Networked energy storage systems require complex control algorithms, circuit designs and high power density energy storage converters
Unlike the essential characteristics of current sources in grid-forming energy storage systems, grid-forming energy storage systems are essentially voltage sources that can internally set voltage parameters to output stable voltage and frequency. Therefore, it is required that grid-forming converters simulate the characteristics of synchronous generators, providing support for voltage and frequency to enhance the stability of the power system. This control strategy necessitates that converters possess higher power density and more complex control algorithms, as well as higher-performance power devices and more intricate circuit designs to implement the control strategy. Effectively managing the heat generated by high power density and complex control strategies, while reducing the size and cost of the cooling system without compromising performance, has become a new challenge in thermal design.
2- Comparison of common cooling solutions
The cooling solution for energy storage inverters has undergone significant iterative evolution in recent years, mainly reflected in the transition of cooling technology from traditional air cooling to liquid cooling technology.
a.Air cooling solution
Air cooling is the temperature control form used in the early stage of energy storage converters. It uses air as the medium and dissipates heat through fans and radiators. The air cooling solution improves heat dissipation efficiency by continuously reducing energy consumption, optimizing structure, and improving heat dissipation materials. At the 2.5MW power level, air cooling can still meet the requirements.
b.Liquid Cooling Solution
As the power density and energy density of energy storage systems continue to increase, liquid-cooled PCS uses coolant with high thermal conductivity as the medium. The coolant is driven by a water pump to circulate in the cold plate and is not affected by factors such as altitude and air pressure. The liquid cooling system has a more efficient heat dissipation efficiency than the air cooling system. The liquid cooling solution has a higher matching degree and has begun to be explored and popularized in the past one or two years.
In addition to the full liquid cooling energy storage solution, some manufacturers have launched energy storage direct cooling machines, which use phase change direct cooling and no water circulation. Direct cooling solutions are also entering the energy storage field.
We will regularly update you on technologies and information related to thermal design and lightweighting, sharing them for your reference.
Thank you for your attention to Walmate.
The battery pack is a key component of new energy vehicles, energy storage cabinets and containers. It is an energy source through the shell envelope, providing power for electric vehicles and providing consumption capacity for energy storage cabinets and containers. In combination with actual engineering needs, this article summarizes the key points of profile design for battery packs by analyzing the requirements of mechanical strength, safety, thermal management and lightweight of battery packs.
1-Battery pack housing design requirements
a.Mechanical strength, vibration resistance and impact resistance. After the test, there should be no mechanical damage, deformation or loosening of the fastening, and the locking mechanism should not be damaged.
b.Sealing: The sealing of the battery pack directly affects the working safety of the battery system. It is usually required to reach IP67 protection level to ensure that the battery pack is sealed and waterproof.
c.The design of the battery pack shell needs to take thermal management performance into consideration and ensure that the battery operates within an appropriate range through appropriate thermal management design.
d.For installation and fixation, the shell should have space for nameplate and safety signs, and reserve sufficient space and fixed foundation for the installation of acquisition lines, various sensor elements, etc.
e.e. All connectors, terminals, and electrical contacts of non-polar basic insulation should meet the corresponding protection level requirements when combined.
f.Lightweighting: Lightweighting of the shell is of great significance to improving the energy density of the battery pack. Aluminum alloy is light in weight and high in quality, making it the most feasible choice at present. The lightweighting level can be improved through appropriate extreme design in combination with actual applications.
g.Durability: The design life of the battery pack shell shall not be less than the service life of the overall product. No obvious plastic deformation should occur during the use cycle. The protection level and insulation performance should not be reduced. The structure should be easy to maintain, including the layout of nameplates and safety signs, and protection of connectors.
Figure 1 Typical aluminum alloy welded battery pack shell
2-Typical aluminum alloy battery pack shell solution
Commonly used aluminum alloy materials for battery pack shells include 6061-T6, 6005A-T6 and 6063-T6, etc. These materials have different yield strengths and tensile strengths to meet different structural requirements. The strength of these materials is: 6061-T6>6005A-T6>6063-T6.
At present, the battery pack shell forming solutions include aluminum profile welding, aluminum alloy casting, cast aluminum plus profile aluminum, stamped aluminum plate welding, etc. The aluminum profile welding solution has become the mainstream choice due to its flexibility and processing convenience. As shown in Figure 1, the shell is mainly composed of an aluminum alloy profile frame and an aluminum alloy profile bottom plate, which are welded using 6 series aluminum alloy extruded profiles. The aluminum alloy casting solution is regarded as the future development direction due to its simplified process and cost reduction potential.
3- Profile section design
a. Section size and complexity: The section size of the profile is measured by the circumscribed circle. The larger the circumscribed circle, the greater the extrusion pressure required. The section of the profile is usually composed of multiple cavities to improve the structural rigidity and strength. Usually, the frame, middle partition, bottom plate, beam, etc. adopt different section designs to adapt to different structural and functional requirements.
Figure 2 Typical aluminum alloy profile section
b. Aluminum profile wall thickness: The minimum wall thickness of a specific aluminum profile is related to the profile circumscribed circle radius, shape and alloy composition. For example, when the wall thickness of 6063 aluminum alloy is 1mm, the wall thickness of 6061 aluminum alloy should be about 1.5mm. The extrusion difficulty of the same section is: 6061-T6>6005A-T6>6063-T6. In the design of battery pack profiles, the frame profile is usually made of 6061-T6 aluminum alloy material, and its typical section is composed of multiple cavities, and the thinnest wall thickness is about 2mm; the bottom plate profile is also composed of multiple cavities, and the material is generally 6061-T6, 6065A-T6, and the thinnest wall thickness is also about 2mm; in addition, in the design of the bottom plate load-bearing tray and bottom plate liquid cooling integration, the bottom plate generally adopts a double-sided structure, the bottom plate thickness is generally 10mm, and the wall thickness and the inner wall of the cavity are about 2mm.
c. Tolerance of profile cross-sectional dimensions: The tolerance of cross-sectional dimensions should be determined based on the processing allowance of the aluminum profile, the conditions of use, the difficulty of profile extrusion, and the shape of the profile. For some aluminum profiles that are difficult to extrude, the shape can be changed or the process allowance and dimensional tolerance can be increased to reduce the difficulty of extrusion and extrude aluminum profile products that are close to the requirements, and then they can be reshaped or processed to meet the use requirements.
In addition, when designing the profile section, it is necessary to consider the specific requirements of different welding processes for joints, grooves, wall thickness, etc.
We will regularly update you on technologies and information related to thermal design and lightweighting, sharing them for your reference.
Thank you for your attention to Walmate.
Battery trays, also known as battery boxes or PACK boxes, are increasingly valued as a very important component in the development of new energy vehicles. The design of battery trays needs to balance the relationship between factors such as weight, safety, cost, and material performance. Aluminum alloys are widely used in automotive lightweight engineering because of their low density and high specific strength, which can ensure rigidity while ensuring vehicle body performance.
1-Battery tray welding location and method selection
Aluminum battery trays are made of extruded aluminum profiles, and the various components are combined into a whole by welding to form a complete frame structure. Similar structures are also widely used in energy storage pack boxes.
The welding parts of the battery tray usually include the bottom plate splicing, the connection between the bottom plate and the side, the connection between the side frame, the horizontal and vertical beams, the welding of liquid cooling system components, and the welding of accessories such as brackets and hanging ears. When selecting welding methods, different welding methods will be selected according to different material and structural requirements, see the table below:
2-Analysis of the influence of welding thermal deformation
Welding is a local heating processing method. Since the heat source is concentrated at the weld, the temperature distribution on the weld is uneven, which eventually leads to welding deformation and welding stress inside the welded structure. Welding thermal deformation is the phenomenon that the shape and size of the welded parts change due to uneven heat input and heat output during the welding process. Combined with actual engineering project experience, the parts that are prone to welding thermal deformation and the influencing factors are summarized:
a. Long straight welding area
In actual production, the bottom plate of the battery tray is generally made of 2 to 4 aluminum alloy profiles spliced together by stir friction welding. The welds are long, and there are also long welds between the bottom plate and the side plate, and between the bottom plate and the spacing beam. Long welds are prone to local overheating in the welding area due to concentrated heat input, resulting in thermal deformation.
Battery tray frame welding
b. Multi-component joints
It is caused by local high temperature heating and subsequent cooling during the welding process at the multi-component weld. During the welding process, the weldment is subjected to uneven heat input, resulting in a significant temperature difference between the weld area and the surrounding parent material, which causes thermal expansion and contraction effects, causing deformation of the welded parts. The electrical installation end of the energy storage pack box is usually equipped with a water nozzle, a wiring harness bracket, a beam, etc., and the welds are dense and very easy to deform.
In the weld-intensive area, the front side of the pallet is warped and deformed
c.Cold plate channel side wall
In the battery tray with integrated design of liquid cooling plate, parts with smaller structural rigidity, such as thin plates and pipe structures, cannot resist thermal deformation well during welding and are prone to deformation. For example, the side wall of the liquid cooling plate flow channel is very thin, generally only about 2mm. When welding beams, wiring harness brackets and other parts on the module mounting surface, it is easy to cause cracks and deformation wrinkles on the side wall of the flow channel, affecting the overall performance.
Thermal crack defects on the liquid cooling channel wall caused by beam welding
3-Welding thermal deformation control method
a. Segment welding, double-sided welding
For parts with relatively low strength requirements, segmented welding is adopted, and the welding process is broken down into multiple small sections. The welds are arranged symmetrically, and the welds are arranged symmetrically near the neutral axis in the construction section, so that the deformations caused by the welds may offset each other. At the same time, the length and number of welds are minimized, and excessive concentration or crossing of welds is avoided, which can reduce the welding temperature gradient and thus reduce welding deformation. For parts with high strength requirements such as the bottom plate, bottom plate and side frame, double-sided welding is adopted to increase strength while reducing bending deformation caused by large parts and long welds.
b.Optimizing welding sequence
Control welding deformation, use joints with lower rigidity, avoid two-way and three-way intersecting welds, and avoid high stress areas. Optimize the welding sequence, weld the weaker rigidity areas first, and the better rigidity areas last, such as welding the fillet welds first, then the short welds, and finally the long welds; weld the transverse welds first, then the longitudinal welds. A reasonable welding sequence can effectively control welding deformation, thereby controlling the weld dimensions.
c. Welding parameter adjustment
Control welding parameters and processes, and reasonably set welding speed, number of welding layers and thickness of each weld. For thicker welds, use multi-layer and multi-channel welding methods, and the thickness of each weld layer should not exceed 4mm. Multi-layer welding can reduce structural microstructure and improve joint performance. Accurately control welding parameters and reasonably select parameters such as welding current, voltage, electrode model and welding speed to ensure consistent shape and size of the molten pool, thereby avoiding errors caused by improper parameter selection.
d.Welding skills improvement
Improve the welder's operating skills (use mechanical processing for large components or nodes with strict requirements) to ensure consistency and standardization of actions during welding and reduce dimensional problems caused by human factors.
We will regularly update you on technologies and information related to thermal design and lightweighting, sharing them for your reference.Thank you for your attention to Walmate.
Aluminum alloy is the most widely used non-ferrous metal structural material in industry, especially in scenarios where the thermal conductivity of materials is of great concern, and in situations where efficient heat conduction is required, such as electronic equipment heat dissipation, electric vehicle three-power heat dissipation, and battery energy storage systems. In the fields of heat dissipation and aerospace, it is usually used to manufacture efficient heat transfer equipment such as radiators, heat conduction plates, and electronic components.
Thermal conductivity, also called thermal conductivity, is a parameter index that characterizes the thermal conductivity of materials. It indicates the heat conduction per unit time, unit area, and negative temperature gradient. The unit is W/m·K or W/m·℃. Aluminum alloy is an alloy material composed of aluminum and other metals. Its thermal conductivity is very excellent, and the thermal conductivity coefficient is usually between 140-200W/(m·K). As the metal with the highest content in the earth's crust, aluminum has a relatively low thermal conductivity coefficient. It is favored because of its high height, low density and low price.
1-Thermal conductivity principle of aluminum alloy materials
When there is a temperature difference between adjacent areas of a material, heat will flow from the high-temperature area to the low-temperature area through the contact part, resulting in heat conduction. There are a large number of free electrons in metal materials. Free electrons can move quickly in the metal and can quickly transfer heat. Lattice vibration is another way of metal heat transfer, but it takes a back seat compared to the free electron transfer method.
Comparison of heat conduction methods between metals and non-metals
2-Factors affecting the thermal conductivity of aluminum alloys
a.Alloying is one of the main factors affecting thermal conductivity. Alloying elements exist in the form of solid solution atoms, precipitated phases and intermediate phases. These forms will bring crystal defects, such as vacancies, dislocations and lattice distortion. These defects will increase the probability of electron scattering, resulting in a reduction in the number of free electrons, thereby reducing the Thermal conductivity of alloys. Different alloying elements produce different degrees of lattice distortion on the Al matrix, and have different effects on thermal conductivity. This difference is the result of multiple factors such as the valence of alloy elements, atomic volume differences, extranuclear electron arrangement, and solidification reaction type.
b.Heat treatment is a very important step in the processing of aluminum alloys. By changing the microstructure and phase transformation of aluminum alloys, its thermal conductivity can be significantly affected. Solid solution treatment is to heat the aluminum alloy to a certain temperature to fully dissolve the solute atoms in the matrix, and then quickly cool it to obtain a uniform solid solution. This treatment improves the material's mechanical properties but usually reduces its thermal conductivity. Aging treatment is through appropriate cold deformation and reheating after solid solution treatment, which can optimize the microstructure of the alloy and improve its overall performance. The aging treatment takes into account the mechanical properties and thermal conductivity of the alloy, so that the alloy maintains high strength while also having good thermal conductivity. Annealing improves the microstructure of the alloy by maintaining it at a lower temperature to precipitate and redistribute the second phase in the alloy. Annealing treatment can improve the plasticity and toughness of aluminum alloys, but the effect on thermal conductivity varies depending on the specific situation.
Schematic diagram of crystal structure changes during the aging process of Al-Cu alloy
c.Other factors influence, impurities and second phase particles: Impurities and second phase particles (such as oxides, carbides, etc.) in aluminum alloys can scatter hot carriers (electrons and phonons), thereby reducing thermal conductivity. The higher the impurity content, the coarser the second phase particles and generally the lower the thermal conductivity. The grain size of aluminum alloys also affects thermal conductivity. Generally speaking, the smaller the grain size, the more grain boundaries there are and the lower the thermal conductivity. In addition, the processing method of aluminum alloy (such as rolling, extrusion, forging, etc.) will affect its microstructure and residual stress state, thereby affecting the thermal conductivity. Work hardening and residual stresses reduce thermal conductivity.
In summary, aluminum alloy is an ideal choice for high thermal conductivity materials. Factors such as the type of alloy elements in aluminum alloys and their forms, heat treatment methods, impurities, grain size, and molding methods will all affect the thermal conductivity of aluminum alloy materials. Comprehensive considerations should be taken when designing material composition and process planning.
We will regularly update you on technologies and information related to thermal design and lightweighting, sharing them for your reference.Thank you for your attention to Walmate.
The air tightness of the battery pack is a key factor in ensuring the quality and safety of the battery pack. It is related to the safety, reliability and service life of the battery pack. The air tightness test of the battery pack should be carried out not only during the production process, but also during battery maintenance and inspection.
1-Battery Pack Airtightness Requirements
In actual production, the air tightness of the battery pack must meet the following requirements:
Sealing performance, the battery pack shell, interface and connectors must have good sealing performance to prevent dust, water vapor and other external impurities from entering the battery pack, which can be achieved through welding, sealants, waterproof materials, etc.
Waterproof performance, to prevent moisture from entering the battery, causing short circuits, corrosion and other problems. According to the national standard GB38031-2020 "Safety Requirements for Power Batteries for Electric Vehicles", the sealing performance of batteries and their components should meet the IP67 standard. Most new energy vehicles have higher sealing performance requirements for batteries and their components, and must meet the IP68 standard, that is, the battery pack can prevent water from entering within the specified water depth and submersion time.
Traditional air tightness testing methods include pressure method and immersion method (water test). The immersion method is to immerse the liquid cooling plate in water and observe whether bubbles are generated to judge the sealing.
Liquid Cooling Plate Water Channel Air Tightness Test Tank
Although the IP68 standard is more stringent, in actual applications, the pressure drop method is often used as the main detection method to meet the IP68 requirements by setting appropriate airtightness detection standards. The pressure drop method determines the airtightness of the battery pack by measuring the pressure change inside the battery pack. When performing airtightness testing, multiple parameters need to be paid attention to, such as inflation pressure, inflation time, pressure stabilization time and leakage rate.
Differential pressure basic principle diagram Direct pressure basic principle diagram
2-Analysis of Liquid Cooling Plate Leakage Problem
With the continuous upgrading of market demand for power battery vehicles, battery energy storage systerms, etc., higher energy density and power density battery packs are widely used. Because of the thermal characteristics of batteries, to ensure the stable operation of core equipment such as batteries and improve energy utilization efficiency, liquid cooling technology is one of the mainstream technical routes for energy storage thermal management, and the air tightness test of the liquid cooling system has become a key link.
Liquid cooling plate leakage is a serious problem: the leakage will hinder the normal flow of the coolant, affect the heat dissipation effect of the liquid cooling plate, and reduce the performance of the equipment; the leakage may also cause aging and damage of system components, reducing the reliability of the system; the leakage may also corrode electronic components and circuits, increasing the risk of equipment failure and fire.
Why does the leakage problem still occur after rigorous air tightness testing during the production and manufacturing process of the liquid cooling plate?
Liquid Cooling System Airtightness Test Process
Liquid seepage may be caused by a variety of factors:
l Tiny cracks and defects. Landscape air tightness testing may detect large leakage channels, but tiny cracks and defects may still exist. These tiny cracks may expand under liquid pressure or high temperature environment, causing liquid seepage.
l Coolant surface tension and wettability differences: When the surface tension of the coolant is low, it is easier to penetrate through tiny gaps. If the surface tension design of the liquid cold plate is unreasonable or the coolant is not properly selected, the liquid seepage problem may be aggravated.
Wettability differences: Different coolants have different wettability on solid surfaces. If the material surface roughness of the liquid cold plate is high or there are microstructural defects, the coolant may penetrate more easily.
l Installation or process problems: If the installation process of the liquid cold plate is not fine enough, or there are defects in the welding, connection and other processes, it may also lead to poor sealing and increase the possibility of liquid seepage.
l Environmental conditions: Changes in temperature, especially in high-pressure environments, may affect the permeability of the coolant. Although these environmental factors may not be considered during air tightness testing, in actual operation, temperature fluctuations may cause seal failure.
l Material aging or fatigue: If the material of the liquid cold plate is used for too long, it may age or fatigue, causing its sealing performance to deteriorate, thereby increasing the risk of liquid leakage.
3-Preventive Measures for Liquid Cooling Plate Leakage
l Improve the design of liquid cooling plate: By optimizing the structure and design of the liquid cooling plate, reduce small cracks and defects, and improve its sealing performance. For example, when welding the module installation beam on the flow channel surface, take anti-leakage measures to avoid coolant leakage.
l Improve the manufacturing process level: In the production process of the liquid cooling plate, high-quality welding processes and materials are used to ensure that the coolant is not easy to penetrate. At the same time, during the assembly process, strictly follow the operating procedures to avoid looseness or incorrect installation.
l Optimize the combination of detection methods to ensure detection efficiency while improving detection accuracy and reducing missed detection rate. The immersion method and pressure drop method are used for air tightness detection, which is simple to operate, economical, and efficient, and is suitable for large-scale routine detection needs. However, the detection accuracy of the two methods is low. The detection accuracy of the pressure drop method is generally a leakage rate of 1×10-4Pa·m³/s, and the accuracy of the detection results is easily interfered by factors such as temperature, humidity, cleanliness, and pressure. Use detection equipment with higher detection accuracy and better effect to increase the detection accuracy to 1×10-6Pa·m³/s, thereby improving the detection effect.
In addition to the preventive measures for the liquid cooling plate itself, it is also necessary to adopt appropriate response strategies in multiple aspects such as coolant selection, seal selection and equipment working environment.
We will regularly update you on technologies and information related to thermal design and lightweighting, sharing them for your reference
Thank you for your attention to Walmate
In heat dissipation design, adopting effective cost-reduction methods can improve the reliability and efficiency of the overall system while reducing unnecessary costs.
1-Derating design reduces costs
Derating design is a design method that intentionally reduces the electrical, thermal and mechanical stresses that components or products are subjected to during operation. In actual production and use scenarios, the stability of electronic equipment can be improved by reducing the stress borne by components.
Schematic diagram of heat dissipation paths for 2D and 3D packaging
l Reduce working stress: During product design and operation, the working stress of components can be reduced by reducing the workload, controlling the operating frequency, limiting the current and voltage, etc.
l Reduce environmental stress: Reduce environmental stress by selecting appropriate component types, layouts, and packaging forms, such as selecting components with a large temperature margin, or using packaging forms with good sealing to reduce the effects of temperature, humidity, and pressure on components.
l Reliability engineering application: reasonable redundant design, fault detection and isolation, etc., further reduce the failure risk of components.
By reducing the stress on components during operation, their power consumption and heat generation can be reduced. When power devices operate under stress conditions lower than their rated stress, their power consumption and heat generation can be reduced, which helps to improve the energy efficiency and reliability of the system. In the long run, derating design effectively increases the life of components, reduces failure rates, reduces maintenance workload, and thus reduces costs.
2-Optimize layout
The working efficiency of the radiator can be significantly improved through reasonable arrangement of thermal components, and a reasonable component layout strategy can achieve a balance between product performance and cost.
l Distribute heat dissipation components: Disperse components that generate large amounts of heat to reduce the heat load per unit area.
l Location that is conducive to heat dissipation: Place the heating element in a location that is conducive to heat dissipation, such as near a vent or the edge of the device.
l Staggered arrangement: During layout, stagger the heating components with other general components, and try to make the heating components principle temperature-sensitive components to reduce their impact on the heat-sensitive components.
l Improve airflow: By changing the direction design and component layout, the airflow path is optimized, the flow rate is increased, and the heat transfer coefficient is improved.
Spacing recommendations between components
3-Choice of cooling method
As the performance of electronic components improves and the degree of integration increases, the power density continues to increase, resulting in a significant increase in the heat generated by the electronic components during operation. When choosing a heat dissipation method for electronic components, the temperature control requirements mainly include the following aspects:
l Temperature range: Different components have different temperature tolerance ranges. For example, high-performance chips such as CPUs have operating temperature requirements between 85-100°C, while some low-power devices can tolerate higher temperatures, so the cooling system must ensure Components operate within a safe temperature range.
l Temperature control accuracy: In some scenarios with strict temperature control requirements, it is necessary to adopt a heat dissipation solution that can accurately control the temperature to avoid component performance degradation or even damage caused by excessively high or low temperatures.
l Ambient temperature: The heat dissipation effect of electronic equipment not only depends on the heat dissipation capacity of the device itself, but is also affected by the surrounding ambient temperature. The heat dissipation design needs to consider changes in ambient temperature and try to keep the device within a suitable temperature range through heat dissipation means.
l Power consumption and reliability: Some low-power electronic components can use natural heat dissipation when they generate low heat. For high-power consumption equipment, it is necessary to wait for the heat dissipation technology of universities to ensure that it maintains normal performance and prolongs operation under high load. service life.
l Sealing and density: In sealed and high-density assembled devices, if the heat generation is not high, you can rely on natural heat dissipation. When components are densely packed and generate large amounts of heat, more effective heat dissipation technologies such as forced heat dissipation or liquid cooling are needed. Liquid cooling and heat pipe technology are used in scenarios with high power consumption and large heat generation, such as high-power electronic components such as traveling wave tubes, magnetrons, and power amplifier tubes, servers and high-power consumption equipment, and three-electric systems of new energy vehicles. Its unique application advantages.
Charging pile air cooling module Charging pile liquid cooling module
When choosing a cooling method for electronic components, it is necessary to comprehensively consider factors such as heat generation and heat flux, ambient temperature and operating temperature, space constraints and thermal isolation requirements, and cost and feasibility. By using appropriate cooling technology and cooling devices to ensure that components operate at a suitable temperature, the cost of system replacement and maintenance can be effectively reduced. In addition, reusing historical projects is also an effective strategy to reduce development and manufacturing costs and improve reliability.
We will regularly update you on technologies and information related to thermal design and lightweighting, sharing them for your reference
Thank you for your attention to Walmate
Energy storage immersion liquid cooling technology is an advanced battery cooling method that uses the efficient thermal conductivity of liquid to achieve rapid, direct and sufficient cooling of the battery, ensuring that the battery operates in a safe and efficient environment. Its basic principle is to completely immerse the energy storage battery in an insulating, non-toxic liquid with heat dissipation capabilities. This technology uses direct contact between the liquid and the battery for heat exchange, thereby quickly absorbing the heat generated by the battery during charging and discharging, and bringing it to the external circulation system for cooling.
Schematic diagram of the principle of a single-phase immersion liquid-cooled energy storage system
As a key component for carrying the battery pack and ensuring that the battery cells work in a suitable environment, the immersion liquid-cooled energy storage Pack box mainly undertakes functions such as carrying the battery pack and coolant, safety protection, and heat conduction. Therefore, in the design of the box structure, it is necessary to comprehensively consider multiple aspects such as airtightness, cooling efficiency, safety, material selection, and processing technology to ensure efficient, safe, and reliable operation of the system. The box structure design is the basis of the entire liquid cooling system.
1-Unified load
The lower box of the immersed liquid-cooled energy storage pack is composed of a bottom plate and side plates. The bottom plate serves as a basic support, and the side plates are fixed around the bottom plate, which together form the main frame of the box. The size of the box should be adjusted taking into account the overall needs and load conditions of the liquid cooling system. In the design of larger-sized boxes, internal partitions or support structures can be reasonably set up to divide the large space into multiple small spaces. force area to improve the uniform load-bearing capacity. In the internal structure, the local load-bearing capacity can be improved by adding support ribs and reinforcing ribs, and a load-sharing structure can also be set up inside the box to balance the load at each corner.
At the same time, in order to reduce the impact of plastic deformation on uniform load-bearing, the processing surfaces of different heights can be designed as the same plane, which can reduce the number of machine tool adjustments and avoid deformation caused by height differences; the width or height of the box can also be increased to disperse the load and reduce deformation.
In addition, the integrated design of the liquid cooling channel and the bottom plate of the box is completed by stir friction welding or laser welding. This design can effectively improve the overall structural strength.
Schematic diagram of the lower box structure of a single-phase immersion liquid-cooled energy storage pack
2-Heat exchange design
Thermal conductivity is an important part of immersion liquid cooling energy storage technology. The design goal is to ensure that the battery can effectively dissipate heat in a high temperature environment, thereby maintaining its performance and safety.
The material of the box should have high thermal conductivity. Commonly used materials are aluminum alloy, copper, and aluminum-based composite materials. The box design also needs to consider the impact of ambient temperature changes. The appropriate thickness of the insulation layer can ensure that the temperature inside the box is within a relatively constant range, thereby improving the overall efficiency of the system.
The structural design of the box directly affects its thermal conductivity. Reasonable flow channel layout ensures that the liquid flows smoothly inside the box and maximizes the contact area, which is the main strategy to improve the thermal conductivity of the box. Multiple flow channels can be set inside the box to increase the coolant circulation path, thereby improving the heat dissipation effect.
Solution 1: single item + plate replacement Solution 2: single item + box replacement
The liquid cooling system includes a cooling medium, a heat-conducting structure, a liquid cooling pipeline and a supporting structure.
In the first scheme, the same or different types of coolants can be selected to be filled into the liquid cooling plate flow channel cavity and the box cavity respectively, and the two cavities are sealed and not connected to each other. In the box cavity, the coolant immerses the battery module, fully contacts it, cools it without flowing, and absorbs the heat on the battery surface by using the good thermal conductivity of the liquid to reduce the temperature rise. In the liquid cooling plate, the coolant is divided into multiple flow channels in the water inlet manifold and enters the cold plate in parallel, and then converges and flows out in the water outlet manifold, which is mainly responsible for taking out the heat and achieving heat dissipation.
In the second scheme, the coolant with low temperature flows in from the bottom or side, and the coolant with high temperature flows out from the top. The coolant circulates in the battery pack, which can effectively and evenly distribute the heat, improve the overall cooling efficiency, and maintain the consistency of the temperature of the battery cell or battery pack.
In order to further improve the cooling effect, a variety of optimization measures can be taken, such as optimizing the liquid flow and circulation method, selecting a coolant with high heat capacity, and improving the temperature distribution of the liquid. These measures reduce heat buildup and energy loss, ensuring the battery operates in an efficiently cooled state.
3-Sealed design
For liquid cooling pack boxes, the fully sealed design is carried out by adopting advanced sealing materials and structures. The sealing design should not only consider air tightness, but also the sealing of liquid media to ensure that the battery cells are leak-free in all directions.
The design should select the appropriate sealing form and shape according to the specific application requirements, and also consider factors such as the leakage freedom, wear resistance, medium and temperature compatibility, and low friction of the seal, and select the appropriate seal type and material according to the detailed specifications.
In addition, the choice of welding process also has a great influence on the sealing performance. For different materials and thicknesses, choosing the appropriate welding method can effectively improve the quality of the weld to ensure the overall strength and sealing of the system.
Finished picture of the lower box of the single-phase immersion liquid-cooled energy storage pack
We will regularly update you on technologies and information related to thermal design and lightweighting, sharing them for your reference.
Thank you for your attention to Walmate.
As the power density of electronic devices continues to rise, heat dissipation design has become a key link affecting system reliability and lifespan. As a professional manufacturer with 19 years of experience in thermal management, we combine typical engineering cases with multi-physics field simulation experience to deeply analyze the five major technical misunderstandings that engineers tend to overlook in high-power scenarios, and provide solutions that conform to industry practices.
Misunderstanding 1: Abuse of high thermal conductivity materials, calculation deviation of interface thermal resistance
Typical problem: Excessive pursuit of theoretical values of thermal conductive materials, ignoring the engineering reality of interface contact thermal resistance.
Case analysis: A certain automotive laser radar heat dissipation module uses a pure copper substrate (thermal conductivity 401W/m·K), but the coating thickness of the thermal interface material (TIM) is not accurately controlled (designed 0.1mm, actual fluctuation ±0.05mm), and the measured contact thermal resistance reaches 0.6°C·cm²/W, causing the FPGA junction temperature to exceed the standard by 22%. After switching to preformed thermal pads (tolerance ±0.02mm, thermal resistance <0.03°C·cm²/W) and combining with vacuum sintering process, the junction temperature is reduced by 17°C, and the MTBF (mean time between failures) is increased to 100,000 hours.
Optimization plan:
Material selection: According to the ASTM D5470 standard, the thermal resistance of TIM is measured, and phase change materials or metal-filled elastomers are preferred.
Process control: Use automatic dispensing equipment (accuracy ±3%) to ensure thickness tolerance <10% and avoid air gaps.
Misunderstanding 2: Mismatch of airflow organization in air cooling system, local hot spots out of control
Typical problem: blindly stacking the number of fans, ignoring the flow channel impedance matching and boundary layer separation effect.
Engineering example: A data center GPU cluster uses 4 12038 fans (air volume 200CFM), but due to improper design of the air guide angle (90° right angle bend), the actual effective air volume is only 65% of the nominal value, and the local temperature difference reaches 40°C. After CFD simulation optimization, the air guide is changed to a gradually expanding flow channel (diffusion angle <7°), and a staggered fin layout is adopted (the spacing gradient increases from 2mm to 3.5mm), the overall wind resistance is reduced by 30%, and the hot spot temperature difference is controlled within 8°C.
Core strategy:
Flow field design: Wind tunnel testing is carried out according to ISO 5801 standard to optimize the inlet and outlet area ratio (recommended 1:1.2).
Dynamic control: Based on the Q-H curve of the PWM fan, the system impedance point is matched to the highest efficiency range (usually 60-80% of the maximum air volume).
Misconception 3: Failure of environmental protection design accelerates material aging
Typical problem: Only focusing on initial heat dissipation performance, ignoring the effects of long-term environmental stress such as humidity, heat, dust, and salt spray.
Case analysis: An offshore wind power converter uses a 6061 aluminum alloy heat sink (surface anodized). After running in a salt spray environment for 6 months, the oxide layer peeled off, causing the contact thermal resistance to increase by 50%, and the IGBT failure rate to surge. Switching to 5052 aluminum alloy (salt spray resistance >1000h) and spraying polyurethane three-proof paint (thickness 50μm, in line with IEC 60068-2-11 standard), the 3-year field failure rate dropped by 75%.
Design points:
Coating technology: Plasma spraying Al2O3 ceramic coating (thermal conductivity 30W/m·K, temperature resistance >800°C) is used in high-temperature scenarios.
Sealing structure: IP69K protection level (double-pass silicone seal + pressure relief valve) to ensure zero intrusion of dust/water vapor.
Misconception 4: Lack of transient heat load modeling and improper selection of phase change materials
Typical problem: The heat dissipation solution designed by steady-state simulation cannot cope with millisecond-level power pulses.
Measured data: A solid-state power amplifier is subjected to a 2ms@2000W pulse load in a 5G base station. The transient temperature rise of the traditional aluminum substrate solution reaches 55°C, while the microcapsule phase change material (paraffin/graphene composite, phase change latent heat 220J/g) combined with transient thermal simulation (ANSYS Icepak transient step length 1ms) is used to control the temperature rise to 18°C, meeting the MIL-STD-810G impact test requirements.
Dynamic management technology:
Phase change packaging: The honeycomb structure is used to encapsulate the phase change material (porosity>70%) to avoid liquid leakage and improve heat diffusion efficiency.
Model calibration: The simulation boundary conditions are corrected based on infrared thermal imaging (sampling rate 100Hz), and the error is <±1.5°C
Misconception 5: TCO evaluation is one-sided and ignores hidden operation and maintenance costs
Typical problem: Only the initial hardware cost is compared, and the energy consumption/maintenance/downtime losses are not quantified.
Cost comparison: A semiconductor factory originally used air-cooled cabinets (initial cost of 500,000 yuan), with an annual electricity bill of 1.2 million yuan (PUE=1.8). After upgrading to two-phase immersion liquid cooling (initial cost of 1.8 million yuan), the annual electricity bill dropped to 450,000 yuan (PUE=1.05), and due to the reduction of clean room air conditioning load, the annual comprehensive savings reached 900,000 yuan, and the incremental investment can be recovered in 2.1 years (IRR>25%).
Decision model:
Refined modeling: Introduce Monte Carlo simulation to quantify the impact of equipment failure rate (Weibull distribution) on TCO.
Policy superposition: Combined with carbon tax mechanisms (such as EU CBAM), the carbon emissions of liquid cooling systems over their entire life cycle are reduced by 60%, and an additional subsidy of 15% is obtained
Conclusion: Upgrading the thermal design paradigm from experience-driven to data-driven
Standardized process: Introduce the JESD51-14 thermal test standard in the conceptual design stage to avoid rework later (the project cycle of a certain optical module is shortened by 30%).
Multidisciplinary collaboration: Through COMSOL Multiphysics coupling electromagnetic loss (HFSS extraction) and thermal strain field, the heat dissipation efficiency of a radar TR component is improved by 40%.
Intelligent operation and maintenance: Deploy fiber grating temperature sensors (accuracy ±0.1°C) and digital twin platforms to achieve fault prediction (unexpected downtime of a supercomputing center is reduced by 90%).
Through rigorous engineering thinking and full-link data verification, thermal design engineers can accurately avoid "invisible killers" and lay the foundation for high-reliability systems. As industry practitioners, we are committed to providing closed-loop solutions from materials to systems, and jointly address thermal management challenges with customers.
We will regularly update you on technologies and information related to thermal design and lightweighting, sharing them for your reference. Thank you for your attention to Walmate.
1- Special tolerance requirements for key parts
In the manufacturing of EV battery trays and Liquid Cooling Energy Storage Battery Pack Enclosure, the tolerance control of key connection surfaces and interfaces directly affects the sealing, heat dissipation efficiency and assembly accuracy.
a. Liquid cooling plate installation surface
The liquid cooling plate is the core component of the battery thermal management system. The flatness of its installation surface directly affects the sealing of the coolant flow channel. If the flatness is out of tolerance, it will cause uneven compression of the silicone gasket, causing local leakage or increased thermal resistance.
b. Welding seam misalignment
The battery tray often uses an aluminum alloy welding structure. Welding seam misalignment will cause stress concentration and reduce fatigue life. Especially for scenarios with high requirements for Battery Pack Enclosure airtightness (such as IP67), misalignment must be strictly controlled.
c. Battery module positioning hole
The positioning hole is used to accurately fix the battery module. Excessive tolerance will cause module misalignment, causing electrical connection failure or mechanical vibration risks.
d. Fixing bolt hole position
The bolt hole is used to connect the Battery Pack Enclosure to the chassis. Position deviation will cause assembly stress and even cause bolt breakage.
e. Battery Pack Enclosure edge straightness
The Battery Pack Enclosure edge straightness affects the assembly of the upper and lower covers and the IP protection level, especially for laser-welded enclosures, where the edge needs to be used as a reference for laser head tracking.
2-The impact of dimensional tolerance on manufacturing efficiency
The impact of dimensional tolerance on manufacturing efficiency is mainly reflected in its balance between processing flow, cost control and product quality. Reasonable tolerance design can not only ensure product function, but also optimize production rhythm. The impact of reasonable control of dimensional tolerance on manufacturing efficiency is mainly reflected in the following aspects:
a. Balance between processing accuracy and production cost
· Although strict dimensional tolerance can improve sealing and assembly accuracy, high-precision processing equipment and complex processes are required, which significantly increases equipment investment and processing time.
· Excessive pursuit of accuracy may lead to increased processing costs. Strict tolerances need to be set in key functional parts, and tolerances should be appropriately relaxed in non-critical areas to reduce costs.
b. Control of rework rate and scrap rate
· Reasonable tolerance design can reduce rework caused by dimensional deviation.
· Welding deformation is the main problem in battery tray manufacturing. The use of CMT cold welding process can optimize heat input, reduce deformation after welding, and shorten the rework cycle.
c. Modular and standardized production
· Through standardized components, tolerance requirements are concentrated on local replaceable parts, reducing the overall processing difficulty.
· Standardized tolerance design can also support multi-model compatibility and reduce the need for customized processing.
3-Industry standards and practical applications of flatness control
For new energy vehicle battery trays, industry standards have strict requirements:
a. Flatness standard: The flatness of the liquid cooling plate installation surface must be ≤0.2mm, the flatness of the bottom support plate must be ≤0.5mm/m², and the flatness error of the frame after welding must be ≤0.8mm6.
b. Manufacturing process optimization: CNC precision milling, CMT cold welding and vibration aging treatment (VSR), combined with laser interferometer online detection, reduce the risk of deformation.
c. Material selection: It is recommended to use high-strength aluminum alloys such as 6061, with a yield strength of ≥1180MPa, taking into account both lightweight and structural stability.
4- Tolerance adjustment strategy driven by materials and processes
a. Material innovation drives lightweighting
Using lightweight high-strength aluminum alloys (such as 6061) and composite materials (silicon carbide reinforced aluminum matrix), combined with anodizing and insulating coating, to achieve 30% weight reduction and improve corrosion resistance, adapting to the heat dissipation requirements of liquid cooling systems.
b. Process collaboration improves manufacturing accuracy
One-piece extrusion molding + stir friction welding (FSW) reduces the number of welds, CMT cold welding and laser welding control thermal deformation.
c. Simulation detection closed loop to ensure reliability
CAE simulation optimizes the layout of reinforcement ribs, laser scanning and helium leak detection technology realize full process monitoring, modular design is compatible with ±5mm tolerance, reducing costs and increasing efficiency.
We will regularly update you on technologies and information related to thermal design and lightweighting, sharing them for your reference. Thank you for your attention to Walmate.
1- Battery tray / energy storage pack box aluminum alloy welding process characteristics
In the manufacturing of new energy liquid-cooled Pack boxes, battery trays and other products, liquid cooling channels and multi-cavity structures are typical complex design features (as shown in Figure 1). Such structures often include the following features:
Figure 1: Typical design of a battery tray
Three-dimensional space interlacing: the flow channels are distributed in a serpentine shape, with a large number of spatial turning points;
Multi-level connection: the main cavity and sub-cavity are connected by thin-walled partitions (thickness 2-3mm);
Miniaturization features: small flow channel cross-section size (the minimum wall thickness of the aluminum profile flow channel is 1.2mm) and poor welding accessibility.
2- Difficulty analysis of combining multiple welding technologies in battery tray manufacturing
In the practice of battery tray manufacturing, it is necessary to combine multiple welding technologies, such as the coordinated application of friction stir welding (FSW), cold metal transition welding (CMT), traditional melting welding (TIG/MIG) and other technologies. This combination aims to take into account lightweight, strength, sealing and production efficiency, but it also faces the following core difficulties:
a. The problem of coordinated heat input of multiple processes
Different welding technologies (such as FSW and laser welding) have large differences in heat input, which can easily cause material performance mutations and thermal deformation, and need to be solved by optimizing the welding sequence and parameter adjustment.
b. Equipment compatibility and efficiency bottleneck
Equipment switching is complex and parameter synchronization accuracy requirements are high. Modular workstation design and intelligent welding machines are required to improve collaborative efficiency and reduce cycle time.
c. Challenges of balancing sealing strength and cost
The risk of leakage at the intersection of multiple welds is high (accounting for 70% of defects), and redundant sealing + topology optimization design is required.
3-Engineering practice: Battery tray, energy storage liquid cooling PACK box multi-welding process coordination
a. Weld scar position planning
· Welding position optimization: In the product design stage, the rationality of the welding position should be fully considered to avoid weld scars appearing in key parts or areas that affect product functions. For example, adjust the gap between the front frame and the water nozzle to prevent weld scar interference; optimize the welding position of the water nozzle to reduce the impact on product performance.
· Matching with product structure: The welding position should match the product structure to ensure that the weld can withstand various loads and stresses during the use of the product. For example, in the liquid cooling plate project, the structural stability and reliability of the product are improved by rationally planning the welding position.
· Easy to operate and detect: The welding position should be convenient for operators to perform welding operations and subsequent quality inspections. For example, avoid welding in locations that are difficult to reach or have poor visibility to improve welding efficiency and quality.
b. Weld scar control
· Weld scar size: The size of the weld scar needs to be strictly controlled. Generally, the weld scar height is required to be within the range of 4/6mm, and the weld scar does not exceed the plane to ensure the appearance and assembly accuracy of the product. For example, in the liquid cooling plate project, the size of the weld scar was successfully controlled by adjusting the welding parameters and process, avoiding the impact of the weld scar on product performance and quality.
· Weld scar shape: The weld scar shape should be as regular as possible to avoid excessive melting or incomplete fusion. By optimizing welding parameters and operating techniques, the weld scar shape can be effectively improved and the weld quality can be improved.
· Grinding treatment: For the concave weld marks produced at the friction welding position, grinding treatment is used to ensure a smooth surface to meet the product appearance and performance requirements.
c. Welding sequence optimization
· Partition welding: For large products, the method of partition welding can effectively control the overall deformation. By dividing the product into several areas and welding them in sequence, the heat concentration during the welding process can be reduced and the risk of thermal deformation can be reduced.
· Step-by-step correction: During the welding process, the step-by-step correction method is used to adjust the welding deformation in time. By continuously measuring and correcting the deformation of the product during the welding process, the dimensional accuracy and shape of the final product are ensured to meet the design requirements.
· Optimize the welding path: Rationally plan the welding path to avoid repeated welding in the same area and reduce heat input. For example, in the liquid cooling plate project, the heat input during the welding process was reduced and the welding quality was improved by optimizing the welding path.
d. Deformation collaborative control
The liquid cooling plate undergoes thermal deformation during the welding process, resulting in a decrease in the dimensional accuracy of the product.
Optimization measures:
· Use partition welding and step-by-step correction methods to control the overall deformation.
· Optimize welding parameters, reduce current and voltage, and reduce heat input.
· Use fixtures to fix the product to reduce movement and deformation during welding.
Use symmetrical welding method to make welding heat evenly distributed.
We will regularly update you on technologies and information related to thermal design and lightweighting, sharing them for your reference. Thank you for your attention to Walmate.
CTC (Cell-to-Chassis) technology has attracted much attention because it can significantly improve the range and space efficiency of electric vehicles, but whether it can truly replace traditional battery technology is still controversial. The core bottleneck on the manufacturing side - the battery tray welding process - has become the key to determining the future of this technology: complex structural design, multi-material compatibility, and stringent thermal management requirements have put traditional welding technology to a huge test. Starting from the perspective of front-line engineers, combining industry trends and engineering practices, this article analyzes the potential and limitations of CTC technology, and explores how welding technology has become the "touchstone" of this technological revolution.
1-The "Ideal and Reality" of CTC Technology
CTC technology can theoretically reduce redundant structural parts and improve energy density by integrating the battery cell directly into the chassis, but the feasibility of its large-scale application is facing realistic challenges:
Car companies' radicalism and caution: Tesla, BYD and other leading companies have launched CTC solutions, but more manufacturers are still waiting and watching. Industry data shows that as of February 2025, CTC mass-produced models account for 15-20% of the world, and traditional CTP (Cell-to-Pack) technology is still the mainstream.
The "cost" of improved battery life: Although CTC can increase energy density by 15%-20%, the complexity of the chassis structure leads to an increase in manufacturing costs of 30%-50% (calculated by a consulting agency), and the balance between cost and benefit is not yet clear.
Maintenance economic controversy: The integrated design has caused the battery maintenance cost to soar. Insurance company data show that the replacement rate of battery assemblies after accidents of CTC models is as high as 70%, far higher than the 25% of traditional models.
These contradictions make the future of CTC technology full of variables. Whether the market can break through 20% depends on three major variables: the progress of Tesla Cybertruck mass production; whether the price war among Chinese automakers forces CTC to reduce costs; and the degree of compromise of European regulations on battery repairability. The level of welding technology on the manufacturing side will be the key to determining whether it can cross the gap between laboratory and mass production.
2-Three "life and death barriers" of welding process
For battery tray manufacturers, the implementation of CTC technology needs to overcome three core problems:
a. The game between "micron-level" precision and efficiency
Traditional welding processes can tolerate 0.5mm errors, but CTC trays need to carry more batteries, and the number of seams increases by more than 3 times, and most of them are distributed on curved and irregular parts. Tests by a manufacturer show that when the welding positioning error exceeds 0.15mm, the yield rate of battery cell assembly will drop sharply from 99% to 82%. What's more tricky is that the improvement in precision is often accompanied by a decrease in efficiency-although the accuracy of laser welding is high, the equipment cost is more than 5 times that of traditional welding.
b. The "water and fire" of mixed materials
In order to balance lightweight and strength, CTC trays often use a mixed structure of "aluminum alloy + carbon fiber + special steel". The difference in thermal expansion coefficients of different materials can be up to 20 times (such as aluminum 23μm/m·K vs. carbon fiber 0.8μm/m·K), which is easy to generate internal stress during welding and cause cracking. When a company tried to connect aluminum and carbon fiber, the crack rate of the weld was as high as 18%, far exceeding the industry's acceptable threshold of 3%.
c. "Invisible killer" of the heat-affected zone
High welding temperature may damage the insulating materials or sensor circuits around the battery cell. A car company once increased the self-discharge rate of the battery module by 50% due to improper control of welding heat input. Engineers must control the welding temperature fluctuation within ±15℃ within 0.1 seconds - this is equivalent to installing a "high-precision temperature brake" on the welding gun.
3-The way out: There is no silver bullet, only system innovation
Faced with these challenges, the industry is exploring three breakthrough paths:
a. "Cocktail therapy" of process combination
Laser arc hybrid welding: Combining the accuracy of laser welding with the advantages of arc welding penetration, the welding speed of aluminum alloy is increased by 40%;
Cold metal transition technology (CMT): Through precise heat input control, the area of heat affected zone is reduced by 60%;
Robot intelligent compensation system: Automatically adjust the welding gun path based on real-time weld scanning data, and increase the qualified rate of complex curved surface welding from 75% to 95%.
b. "Pre-compromise" on the material side
Some companies have begun to jointly develop "welding-friendly" composite materials with material suppliers. For example, the welding crack sensitivity of a domestic modified aluminum alloy (aluminum alloy material adjusted by physical, chemical or process means) has been reduced from level 7 to level 3 (according to ISO standards). Although it sacrifices 5% of the lightweight effect, it increases the welding yield to 98%.
4- Engineers’ new proposition: Finding certainty in uncertainty
a. The controversy over CTC technology is essentially a game between “system optimization” and “local limits”:
If the breakthrough speed of welding process is slower than the cost reduction expectations of car companies, CTC may become a niche technology;
If materials, processes, and testing technologies make breakthroughs in a coordinated manner, it is expected to usher in a new era of electric vehicle structural design.
b. For engineers, it is necessary to reconstruct capabilities from two dimensions:
Cross-domain knowledge integration: understand the sensitive threshold of electrochemical properties to welding heat input;
Agile response capability: A case study of a European supplier shows that the team that can complete the optimization of welding parameters of new alloy materials within a week has a 3-fold increase in the probability of obtaining orders.
CTC technology stands at the crossroads of “disruptive innovation” and “mass production trap”. It may not completely replace existing technology, but it is forcing the welding process to evolve towards higher precision, stronger compatibility, and more intelligent control. In this technology marathon, the real winner may not be the earliest car company, but the engineering team that turns “impossible welds” into “standardized interfaces” on the manufacturing side.
We will regularly update you on technologies and information related to thermal design and lightweighting, sharing them for your reference. Thank you for your attention to Walmate.
As CTP (Cell to Pack) technology completely subverts the traditional battery pack structure, the "role" of the battery tray has shifted from passive load-bearing to active integration. Welding technology has become the core of safety and performance. The requirements of lightweight (wall thickness is only 1.5mm), zero pore sealing, and multi-material (aluminum/copper/carbon fiber) integration have caused traditional welding to fall into the dilemma of deformation and defects. The industry is making breakthroughs through material innovation, intelligent quality inspection and process iteration. This article will analyze the disruptive challenges that CTP poses to welding and explore the technical path to high precision and high reliability.
1-Analysis of the changes in the structural design requirements of battery trays due to CTP technology
CTP (Cell to Pack) technology directly integrates the battery cells into the battery pack by eliminating the module structure in the traditional battery pack. This technological innovation puts forward all-round and multi-dimensional upgrade requirements for the structural design of the battery tray. The following is a specific analysis from the perspectives of materials, performance, process, integration, etc.:
(1) Comprehensive improvement of structural strength and shockproof performance
a. Mechanical bearing requirements after eliminating the module:
After CTP technology eliminates the module structure, the battery tray needs to directly bear the support, fixation and external force buffering functions of the battery cell. The traditional module disperses the mechanical load, while the CTP tray needs to absorb the expansion deformation of the battery cell during charging and discharging as a whole (for example, the expansion force of the square battery cell can reach 10-20kN), while resisting the vibration, extrusion and impact load during vehicle driving.
b. Material and structural optimization direction
· Dominance of high-strength aluminum alloy: Early steel was gradually replaced due to its heavy weight, and 6061-T6 became the mainstream. It has high specific strength and strong corrosion resistance, which can meet the dual requirements of lightweight and high rigidity.
· Composite structure design: For example, the "double-frame ring beam" structure of Leapmotor enhances local impact resistance through longitudinal and transverse beam compartments, while using extrusion technology to optimize material distribution and reduce redundant weight.
· Exploration of magnesium-aluminum alloy and carbon fiber: Magnesium-aluminum alloy is 30% lighter than traditional aluminum materials, and carbon fiber composite materials have both high strength and lightweight characteristics, but are currently only used in high-end models due to cost and process maturity.
(2) Requirements for integrated air tightness and thermal management
a. Sealing performance upgrade
After the module is removed, the internal coolant circulation and gas sealing of the battery pack are completely dependent on the tray, and welding defects (such as pores and cracks) may lead to leakage risks.
Figure 1-Battery tray air tightness test
b. Integration of thermal management functions
CTP trays need to integrate components such as liquid cooling plates and thermal conductive adhesives. For example, structural adhesives are used to fix the battery cells and transfer expansion stress, and polyurethane thermal conductive adhesives (thermal conductivity > 1.5 W/m·K) are used for heat conduction between battery cells and with liquid cooling tubes. The amount of adhesive used in a single PACK is more than 50% higher than that in traditional structures. Flow channels need to be designed inside the tray to optimize cooling efficiency while avoiding damage to the sealing caused by the heat affected zone of welding.
(3) Lightweighting and material innovation
a. Material selection trends
Aluminum alloy extrusion and die-casting process: extruded aluminum profiles are used for frame structures (such as Tesla's 4680 battery tray), and die-casting processes (such as integrated die-casting) simplify the welding process and reduce weight by 15%-20%.
Application of plastic composite materials: For example, glass fiber reinforced PA6 materials are used in non-load-bearing parts to further reduce weight, but the compatibility problem with the metal connection interface needs to be solved.
b. Lightweight design strategy
Topology optimization: Reduce redundant materials through CAE simulation and reduce the weight of the tray while ensuring strength.
Thin-wall and integrated: The wall thickness of the tray is reduced from 3mm to 1.5-2mm, and functional components such as BMS brackets and wiring harness channels are integrated to reduce the number of parts.
(4) Integration and modular design
a. High integration of functional components
CTP trays need to integrate modules such as battery management system (BMS), high-voltage connectors, and fireproof isolation layers.
b. Modular and compatible design
The welding production line needs to support mixed production of multiple models of trays, be able to achieve "one-click change of type", and be compatible with tray structures of different battery cell sizes (such as square and cylindrical).
2-Specific challenges of CTP technology innovation to welding process
CTP (Cell to Pack) technology has significantly improved space utilization and energy density, but it has also posed unprecedented challenges to welding process.
(1) The difficulty of controlling welding defects has increased sharply
a. Porosity and sealing challenges
After CTP technology eliminates the module, the battery tray needs to directly assume the sealing function. Welding pores (a common defect in aluminum alloy welding) will directly lead to the risk of coolant leakage or gas penetration.
b. Cracks and material compatibility
High zinc aluminum alloys (such as 7 series) are prone to cracks due to thermal stress during welding.
c. Gap and assembly error
The direct integration of multiple cells leads to an increase in the number of splicing points of the tray structure. The accumulation of assembly errors may cause the weld gap to exceed ±1mm.
(2) Process adaptation problems brought about by the upgrade of the material system
a. Welding challenges of lightweight materials
The CTP tray material has shifted from steel to aluminum alloy (6061-T6, 7075-T6), magnesium-aluminum alloy (30% weight reduction) and carbon fiber composite materials. Aluminum alloy welding needs to solve the problem of insufficient penetration caused by the difficult melting of the oxide film and high thermal conductivity.
b. Dissimilar material connection technology
When the tray integrates functional components such as liquid cooling plate (copper/aluminum) and fireproof layer (ceramic matrix composite material), brittle intermetallic compounds are prone to appear at the interface of dissimilar materials.
(3) Upgraded structural complexity and precision requirements
a. Deformation control of large-size thin-walled structures
The wall thickness of the CTP tray is reduced from 3mm to 1.5-2mm, and the sensitivity of welding thermal deformation increases sharply.
b. High-density solder joints and process efficiency
The number of solder joints on a single tray has increased from 2,000 in traditional modules to more than 5,000.
Figure 2- Battery tray welding
3-Upgrade of production process and quality control
CTP technology promotes the transformation of battery tray welding from "single process" to "multi-technology collaboration, intelligence, and greening". Manufacturers need to focus on three major directions:
Technology upgrade: break through the control of defects such as pores and cracks, and adapt to lightweight materials;
Intelligent transition: full process digitization and AI quality inspection to achieve high-precision production;
Ecological collaboration: jointly build technical standards with material suppliers, equipment suppliers, and OEMs.
We will regularly update you on technologies and information related to thermal design and lightweighting, sharing them for your reference. Thank you for your attention to Walmate.
Lightweight materials like aluminum alloys have become mainstream due to their superior performance. However, challenges such as heat input control, deformation suppression, and process stability in thin-plate welding pose significant hurdles for traditional welding technologies. Cold Metal Transfer (CMT) welding, with its advantages of low heat input, spatter-free transfer, and intelligent parameter control, offers an innovative solution for battery tray manufacturing.
This article delves into precision control strategies of CMT technology in thin-plate welding for battery trays, analyzing its adaptability, process challenges, and composite application scenarios, aiming to provide theoretical and practical guidance for efficient, high-quality production.
Figure 1: CMT Welding of 104S Energy Storage Battery Liquid Cooling Lower Enclosure
1-Battery Tray Welding Requirements and CMT Adaptability
CMT technology, with its low heat input, spatter-free transfer, and intelligent parameter control, perfectly aligns with the high precision, low deformation, and efficiency demands of battery tray welding.
(1)Core Process Requirements for Battery Tray Welding
a. Material Compatibility and Lightweighting Needs
Battery trays primarily use lightweight aluminum alloys (e.g., 6xxx series, 6061) or carbon fiber composites for high-end models, requiring high strength (60%–70% base material tensile strength) and low density (aluminum alloy: 2.7g/cm³).
Dissimilar Material Joining: For hybrid steel-aluminum structures, thermal expansion coefficient differences must be addressed to minimize deformation.
b. Weld Quality and Performance Metrics
Low Heat Input and Deformation Control: For thin plates (0.3–3mm), deformation must be ≤2mm. Long linear welds require segmented welding or anti-deformation design.
Sealing and Strength: Welds must be fully sealed to prevent electrolyte leakage and pass shear tests (e.g., T/CWAN 0027-2022 standards).
Porosity Control: Aluminum alloy welding is prone to porosity, requiring a porosity rate ≤0.5%.
c. Production Efficiency and Automation Requirements
Batch production demands a welding speed ≥7mm/s, reducing single-tray welding time to 5–10 minutes.
Automated workstations must support dual-station design (simultaneous assembly and welding) and multi-robot collaboration.
(2)Key Advantages of CMT for Battery Tray Welding
a. Precise Low Heat Input Control
CMT reduces heat input by 33% compared to traditional MIG welding by retracting the welding wire to cut off current during droplet short-circuiting, eliminating burn-through risks for ultra-thin plates (0.3mm).
Alternating cold-hot cycles (arc heating-droplet transfer-wire retraction) minimize heat accumulation, controlling deformation to ≤1.5mm (BYD and BAIC case studies).
b. Process Stability and Quality Enhancement
Spatter-Free Welding: Mechanical retraction eliminates droplet spatter, reducing rework.
Porosity Optimization: Using Ar+30%He shielding gas reduces porosity by 50% compared to pure Ar, with pore sizes ≤0.3mm.
High Gap Tolerance: Accommodates assembly gaps up to 1.5mm, lowering fixture precision requirements.
c. Automation Integration and Efficiency Gains
Dual-station workstations (e.g., Taixiang Tech designs) enable parallel welding and assembly, doubling efficiency.
Symmetric robot welding (dual-robot synchronization) with anti-deformation design reduces cycle time to ≤10 minutes.
2-Challenges in CMT Process for Battery Trays
Figure 2: CMT Welding Process Flow
(1)Material Properties and Weld Defect Control
a. Porosity Sensitivity in Aluminum Alloy Welding
Aluminum alloy trays (e.g., 6061, 6063) are prone to porosity due to rapid solidification and hydrogen solubility changes. Shielding gas composition is critical: pure Ar results in ~5% porosity, while Ar+30%He reduces porosity to ≤0.5%. Inductance adjustment (e.g., negative tuning) optimizes molten pool flow, minimizing pore size.
b. Hot Cracking and Composition Segregation
Segregation of Mg, Si, etc., in aluminum alloys can cause grain boundary embrittlement. While CMT’s low heat input reduces HAZ, precise control of welding speed and wire feed is needed to avoid insufficient penetration or localized overheating.
c. Metallurgical Compatibility in Dissimilar Material Welding
Interfaces in Al-steel or Al-composite joints (e.g., crash beams and enclosures) must mitigate brittle phases (e.g., FeAl₃) and Zn vapor interference.
(2)Process Parameter Optimization Challenges
a. Balancing Penetration and Heat Input
Welds must meet T/CWAN 0027 standards for penetration depth (≥0.8mm). CMT’s low heat input may lead to insufficient penetration, requiring arc length adjustment or pulsed current to enhance penetration.
b. Trade-off Between Speed and Stability
Automated lines demand speeds ≥1.2m/min, but high speeds risk arc instability or uneven droplet transfer.
c. Gap Bridging in Complex Welds
Trays often feature large gaps (0.5–1.5mm) or irregular joints (e.g., T-joints).
(3) Compatibility of structural design and manufacturing process
a. Thin plate welding deformation control
The wall thickness of aluminum alloy pallets is usually 2-3mm. The deformation of traditional MAG welding can reach 1.2mm, while CMT welding can reduce the deformation to less than 0.3mm through low heat input. However, it is necessary to cooperate with anti-deformation tooling design and robot symmetrical welding (double-station workstation) to further improve the accuracy.
b. Continuity and sealing of long welds
The length of the sealed weld of the battery tray can reach several meters, and arc breaking or molten pool fluctuations must be avoided. CMT technology ensures the uniformity of the weld through more than 70 arc reignition cycles per second, and the airtightness qualification rate can be increased to 99% with the laser tracking system.
c. Synergy of multi-process composite applications
High-end pallets often use CMT+FSW (friction stir welding) composite process: CMT is used for complex structures (such as the connection between the frame and the bottom plate). FSW is used in high-load areas (such as longitudinal beams) to improve strength. The matching problem of the connection parameters of the two processes (such as preheating temperature and post-weld heat treatment) needs to be solved.
3-Typical application scenarios of CMT process in battery tray manufacturing
(1) Connection of the main structure of the battery tray
a. Frame and bottom plate welding
CMT process is widely used in the connection between the frame and the bottom plate of aluminum alloy battery trays, especially for long welds and thin plates (2-3mm thickness)
b. Connection between beam and bottom plate
In the design of CTP battery trays, due to the reduced number of beams and complex structure, CMT process is used for: High-precision positioning welding: The local connection between the beam and the bottom plate (such as T-joint) needs to avoid insufficient penetration. CMT achieves stable penetration ≥ 0.8mm through digital arc length control (such as Fonis CMT Advanced technology). Multi-material adaptation: If the beam is made of aluminum-magnesium alloy (such as 6061) and the bottom plate is high-strength aluminum, CMT can reduce pores through Ar+He mixed gas protection, while adapting to the thermal conductivity differences of different materials.
(2) Welding of thin plates and complex geometric structures
a. Thin-wall aluminum alloy welding (2-3mm)
The lightweight demand for battery trays promotes the application of thin plates, but traditional MIG welding is prone to deformation. The advantages of the CMT process are:
b. Ultra-thin plate welding: Taixiang Automation uses CMT technology to achieve spatter-free welding of 0.3mm ultra-thin plates for battery tray edge sealing structures.
c. Bridging of special-shaped welds: For special-shaped structures such as internal reinforcement ribs and anti-collision beams of the tray, the CMT Gap Bridging mode can fill the 0.5-1.5mm gap through wire retraction and arc redirection to avoid unfused defects.
d. Welds with high sealing requirements: The sealing of the battery tray is directly related to battery safety. The CMT process ensures it in the following ways:
· Continuous long welds: Using more than 70 arc reignition cycles per second (such as Fronius LaserHybrid technology) to ensure the continuity of several meters of welds, with an airtight pass rate of 99%.
· Low heat input control: Compared with laser welding, CMT has lower heat input, which reduces the thermal impact of molten pool fluctuations on the sealant layer and is suitable for the glue coating process.
(3) Multi-process composite manufacturing scenario
a. CMT+FSW composite process
In high-end battery tray production lines, CMT is often coordinated with friction stir welding (FSW):
Division of labor and cooperation: CMT is used for flexible welding of complex structures (such as frames and special-shaped joints), and FSW is used for high-load areas (such as longitudinal beams) to improve strength. For example, Shanghai Weisheng's automated production line uses a combination of CMT+FSW+CNC to increase the tray production efficiency by 30%.
Process connection optimization: Huashu Jinming's production line adopts a modular design, and achieves seamless connection with FSW through preheating parameter matching (such as local heating to 150°C after CMT welding).
b. Combined with FDS/SPR riveting technology
In the second-generation CTP technology, CMT is coordinated with friction self-tightening (FDS) and self-piercing riveting (SPR) technology: Hybrid connection solution: For example, the load-bearing area of the frame and the bottom plate adopts FSW, while the detachable parts (such as water-cooling plates and insulation layers) are pre-positioned by CMT welding and then fixed by FDS riveting, taking into account both strength and maintenance convenience.
The explosive growth of new energy vehicles and energy storage has positioned battery tray welding technology at the core of manufacturing processes. Facing the dual challenges of aluminum alloy lightweighting and complex structures, this article delves into battery tray welding technologies, comparing the principles, performance metrics, and application scenarios of conventional fusion welding, friction stir welding (FSW), and laser welding. Through multi-dimensional analyses of heat-affected zones (HAZ), joint strength, corrosion resistance, and more, we present a comprehensive evaluation of the advantages and disadvantages of these welding technologies.
1-Technical Principle Comparison
a. Conventional Fusion Welding
Principle: Uses heat sources such as electric arcs or plasma arcs to locally melt the welding joint, forming a molten pool that solidifies into a weld upon cooling. Protective gases (e.g., CO₂, argon) or flux are required to prevent oxidation, and filler materials such as wires or rods may be added.
Characteristics: High molten pool temperatures and rapid cooling lead to coarse columnar grains. The heat-affected zone (HAZ) is wide, with insufficient metallurgical processes, resulting in defects like pores and cracks.
b. Friction Stir Welding (FSW)
Principle: Utilizes a high-speed rotating tool to generate frictional heat with the workpiece, bringing the material to a thermoplastic state. Mechanical stirring and plastic flow achieve solid-phase bonding without forming a molten pool or requiring filler materials.
Characteristics: Welding temperatures remain below 80% of the material’s melting point. Dynamic recrystallization forms fine-grained structures, resulting in dense, defect-free welds with low heat input and minimal deformation.
c. Laser Welding
Principle: A high-energy-density laser beam is focused on the workpiece surface. Heat conduction (power density <10⁵ W/cm²) or deep-penetration welding (power density ≥10⁵ W/cm², forming a keyhole effect) achieves material melting and bonding.
Characteristics: Extremely narrow HAZ, deep penetration, high welding speed, and precision capabilities. However, it is sensitive to material surface reflectivity and requires strict control of process parameters.
a- Conventional Fusion Welding b- Friction Stir Welding c- Laser Welding
Figure 1: Principles of Common Battery Tray Welding Technologies
2-Performance Metrics
a. Heat-Affected Zone (HAZ) Comparison
Key Analysis:
Conventional Fusion Welding: High heat input leads to wide HAZ, grain coarsening, and metallurgical defects (e.g., pores), significantly reducing material performance.
FSW: Solid-phase bonding avoids molten pools. HAZ is divided into TMAZ and HAZ, with coexisting fine grains (NZ) and localized deformation (TMAZ).
Laser Welding: Ultra-narrow HAZ (0.1–0.5 mm) due to high energy density and rapid cooling, but keyhole effects may impact microstructural uniformity.
b. Joint Strength Comparison
Key Analysis:
Conventional Fusion Welding: Rapid solidification causes coarse grains and defects, significantly reducing joint strength. For example, MIG-welded aluminum alloys exhibit only 72.8% of the base material’s tensile strength.
FSW: Dynamic recrystallization forms fine grains (NZ), but TMAZ grain deformation and HAZ phase dissolution may create weak zones.
Laser Welding: High cooling rates suppress grain coarsening, yielding weld strength close to the base material.
c. Corrosion Resistance Comparison
Key Analysis:
Conventional Fusion Welding: Coarse grains and defects lead to preferential corrosion in HAZ and fusion lines.
FSW: NZ exhibits superior corrosion resistance due to fine grains and homogenization, but HAZ grain coarsening and secondary phase precipitation (e.g., Fe-containing phases) may create corrosion-sensitive zones.
Laser Welding: Narrow HAZ and uniform microstructure reduce active corrosion sites, but surface oxidation layers must be managed for optimal corrosion resistance.
3-Application Scenarios
a. Conventional Fusion Welding
· Applicable Areas:
Frame-to-Baseplate Connections: Used for welding the main structure of battery trays, such as aluminum profiles in BYD and BAIC models.
Edge Beams and Small Component Repair Welding: Suitable for complex or spatially restricted areas (e.g., edge beams, stiffeners) requiring supplementary welding with other processes (e.g., FSW).
· Suitable Workpieces:
Thick plates (e.g., steel trays or thick aluminum plates).
Non-sealed auxiliary structures (e.g., battery pack corner fixtures).
· Suitable Materials:
Aluminum Alloys: 6xxx series (e.g., 6061-T6) for thick plates, though joint strength is low (70%–80% of base material).
Steels: Cost-effective but heavy for steel battery tray frames.
· Limitations: High heat input causes significant deformation, unsuitable for high-precision or thin-plate welding.
b-Friction Stir Welding (FSW)
· Applicable Areas:
Baseplate Splicing: Long-seam splicing for aluminum trays, e.g., integrated cooling channel structures (Guangdong Walmate Tech case).
High-Sealing Zones: Battery tray-to-enclosure joints (e.g., Geely and XPeng models use double-sided FSW for sealing).
Complex Profile Connections: T-joints or hollow profiles (self-supporting via dual-shoulder FSW).
· Suitable Workpieces:
Aluminum extruded profiles (e.g., 10mm-thick baseplates with 2mm wall double-layer designs).
Dissimilar material welding (Al/Cu, Al/Mg), requiring specialized process optimization.
· Suitable Materials:
Aluminum Alloys: 6xxx series (6061-T6, 6005A-T6, 6063-T6), achieving 80%–90% base material strength.
Magnesium Alloys: Lightweight trays (e.g., Ruixiang Tech case), but heat input must be controlled to prevent grain coarsening.
· Technical Advantages: Solid-phase bonding avoids fusion defects, ideal for lightweight and high-sealing applications.
c-Laser Welding
· Applicable Areas:
High-Strength Steel Tray Critical Welds: High-stress zones (e.g., battery tray-to-body connections).
Precision Seal Welding: Cover plate seal welding (up to 40 mm/s speed with minimal HAZ).
Thin-Plate Splicing: Efficient splicing for thin aluminum/steel plates (<3mm), minimizing deformation.
· Suitable Workpieces:
High-precision, automated production lines (e.g., robotic laser welding systems).
Complex geometries (e.g., curved seams) requiring dynamic positioning systems.
· Suitable Materials:
High-Strength Steels: Tensile strength ≥1000 MPa, retaining 95% of base material strength.
Aluminum Alloys: Require surface pretreatment (e.g., anodizing) to reduce reflectivity, increasing costs.
· Limitations: Multi-pass welding for thick plates (>8mm aluminum), lower efficiency than FSW.
We will regularly update you on technologies and information related to thermal design and lightweighting, sharing them for your reference. Thank you for your attention to Walmate.
As a front-line engineer at a battery tray manufacturer, I have participated in multiple new energy vehicle battery shell projects and have a deep understanding of the industry's game in the triangle relationship of "lightweight-safety-cost". This article will analyze the selection logic and industrialization challenges of the current technology route from three dimensions: material route, manufacturing process and future trends, combined with actual cases.
1-Material route: the trade-off between lightweight and cost
The choice of battery shell material directly affects the performance and economy of the whole vehicle. The current mainstream routes can be summarized into three categories: aluminum alloy, high-strength steel and composite materials, each with its own applicable scenarios.
a. Aluminum alloy route: the main force of lightweight
· Extruded aluminum profiles: BYD's battery shells of many models are made of extruded aluminum profiles, which achieve weight reduction by optimizing the cross-sectional shape and improve structural rigidity.
· Die-casting integration: Tesla's 4680 battery is combined with CTC technology to reduce weight by more than 50%, but the mold cost exceeds 200 million yuan, and an annual production of more than 500,000 pieces is required to dilute the cost, which is suitable for large-scale production of leading car companies.
· Composite aluminum: Porsche Taycan adopts an aluminum-carbon fiber hybrid structure, which further reduces weight by 15%, but the cost increases by 30%, which is limited to high-end models.
b. High-strength steel route: counterattack in the cost-sensitive market
Hot-formed steel (such as 22MnB5) has a yield strength of 1500MPa, a penetration rate of over 40% in commercial vehicles, and a single GWh cost that is 40% lower than aluminum, but the energy density is sacrificed by about 8%. Therefore, high-strength steel is generally used in models below 100,000 yuan, taking into account both cost and basic safety.
c. Composite materials: a trial of high-end
· SMC material: The upper shell of the battery pack uses glass fiber reinforced composite materials, which is 30% lighter than steel, but the impact resistance shortcomings need to be compensated by increasing the thickness (3mm+5mm reinforcement).
· Carbon fiber: The shell of the BMW i3 is 50% lighter than aluminum, but the cost is as high as 800 yuan/kg, and it is difficult to mass produce. It is currently only used for luxury models.
Practice summary: Material selection needs to match the model positioning. The mid-range market (200,000-300,000 yuan) is mainly aluminum-based materials, the low-end market relies on high-strength steel, and luxury models explore carbon fiber composite solutions.
2- Manufacturing process: the balance between efficiency and reliability
The manufacturing process of the battery shell directly affects the production efficiency and product reliability. The current mainstream technical routes include die casting, extrusion welding and structural integration technology.
a. Die casting vs. extrusion welding
· Extruded aluminum profiles (used by GM Bolt): The cost of a single piece is about 800 yuan, which is suitable for mass production, but the process is complicated.
· Die-cast aluminum (NIO ET5): The cost of a single piece is 1,500 yuan, but the production efficiency is increased by 5 times, which is suitable for rapid production demand.
· Friction stir welding (FSW): Compared with traditional arc welding, the deformation is reduced by 50%, and the fatigue resistance is improved by 30%, but the equipment investment needs to be increased by 40%, which is suitable for commercial vehicles with high life requirements.
b. Structural integration technology
· CTP modularization (CATL Kirin battery): The space utilization rate is increased from 72% to 85%, and the cost is reduced by 15-20%, but the thermal runaway protection design needs to be strengthened.
· CTC chassis integration (Tesla Model Y): 370 parts are reduced and the battery life is increased by 54%, but the maintenance cost increases by 300%, posing a challenge to the after-sales system.
Key data comparison
Production line experience: CTP technology is still the current mainstream due to its high compatibility; while CTC requires in-depth collaboration between car companies and battery manufacturers and is unlikely to be popularized in the short term.
3- Future trends: technology integration and intelligent upgrade
a. Material composite
Aluminum/carbon fiber hybrid shell (such as Porsche Taycan concept) can reduce weight by 15% and achieve 800MPa compressive strength, but the interface bonding strength needs to be greater than 25MPa (Toyota bZ4X mass production qualification rate is only 65%), and process stability needs to be broken through.
b. Functional integration innovation
· Liquid cooling plate and shell integration (GAC magazine battery): cooling contact area increased by 50%, temperature difference control <5℃, but aluminum-copper dissimilar welding porosity needs to be optimized by laser swing welding.
· Embedded fiber optic sensor (Continental Group solution): real-time monitoring of shell strain and temperature, BMS response speed increased by 30%, but the sensor durability problem needs to be solved.
c. Green circulation system
BMW's closed-loop recycling of recycled aluminum technology reduces carbon emissions by 60%, but the performance loss of recycled materials needs to be controlled within 10%. In addition, large thin-wall die casting (such as LK 9000T die casting machine) requires precise control of mold temperature difference (±5℃), and burr cleaning efficiency becomes a bottleneck for mass production.
Process breakthrough direction:
· Compression of composite material molding cycle (such as LGF-PP injection molding cycle needs to be shortened from 180 seconds to 90 seconds);
· Application of digital twin technology to reduce collision simulation error from ±20% to ±5%, improving design reliability.
4- Market stratification and industrialization outlook
Short term (1-3 years): CTP+ extruded aluminum profiles are still the mainstream, and CATL continues to lead with a 34% market share;
Medium term (5-10 years): CTC and carbon fiber are accelerating their penetration in the high-end market, and it is expected that carbon fiber will account for 25% in 2030;
Long-term goal: Energy density will break through 400Wh/kg, and at the same time, based on the national standard 30-minute thermal runaway protection, further improve safety redundancy.
5-Conclusion
The essence of choosing the technical route of battery housing is "scenario-based adaptation", which requires comprehensive consideration of the positioning of car companies, cost thresholds and supply chain maturity. As engineers, we must embrace innovation in materials and processes, and also focus on the feasibility of mass production, and find the optimal solution in the dynamic balance between lightweight, safety and cost. In the future, with the maturity of intelligent and green manufacturing technologies, battery housings will gradually shift from "passive protection" to "active safety", providing solid support for the full popularization of new energy vehicles.
We will regularly update you on technologies and information related to thermal design and lightweighting, sharing them for your reference. Thank you for your attention to Walmate.
In the actual production, assembly and use process, the insulation withstand voltage failure of battery trays often occurs, like a reef hidden in the rapid development of the new energy industry, threatening the driving safety of vehicles and the reliable operation of the energy storage system. This article attempts to analyze the typical failure modes based on manufacturing practice, in order to provide some superficial references for relevant practitioners and help industry colleagues jointly explore effective ways to improve the insulation withstand voltage performance of battery trays.
Part 3 -Common failure analysis
Figure 1 Insulation withstand voltage test
1- Analysis of common failure mechanisms
The application scenarios of battery trays for energy storage and new energy vehicles are different, resulting in both commonalities and differences in insulation withstand voltage requirements and failure conditions, as follows:
a. Differences
Dynamic load: New energy vehicles need to deal with high-frequency mechanical vibrations of 20-2000Hz, while energy storage systems mainly face long-term static deformation, with cumulative deformation time exceeding 10 years.
Voltage level: The insulation withstand voltage of new energy vehicle battery trays must reach 3000VDC and above (based on GB 38031), while energy storage systems require insulation withstand voltage of 4200VAC and above (based on IEC 61439).
Failure acceleration factor: New energy vehicles generate thermal stress due to more than 2000 charge and discharge cycles per year, which accelerates insulation failure; energy storage systems operate 24/7, with an average annual operating time of more than 8000 hours, resulting in electrochemical aging, which in turn accelerates insulation failure.
b. Common points
Both need to achieve early warning of insulation failure through insulation resistance monitoring (not less than 100MΩ in cold state and not less than 1MΩ/kV in hot state) and partial discharge control (less than 5pC).
2- Common manufacturing defects that cause insulation withstand voltage failure of battery trays
(1) Insulation withstand voltage construction stage
a. Potential failure and cause analysis
· Material defects:
Cause: Insulation material withstand voltage level is insufficient, aging due to moisture or surface contamination (oil stains, metal debris).
Performance: Low insulation resistance (<100MΩ), leakage current exceeds the standard or direct breakdown during withstand voltage test.
· Process problems:
Cause: Residual metal debris (rough welding/cutting process), uneven thickness of insulation coating or uncured.
Performance: Partial discharge, insulation layer damage causes short circuit.
· Environmental interference:
Cause: High temperature and high humidity cause material moisture absorption and chemical corrosion.
Performance: Insulation performance deteriorates with the environment, and condensed water causes creepage.
· Test errors:
Cause: Wrong test voltage/time setting (such as not in accordance with GB/T 38661 standard), poor grounding.
Performance: Misjudgment of qualified or overvoltage damage to components.
b. Response strategy
· Material optimization: Select moisture-proof materials (such as carbon fiber composite materials) with a withstand voltage of ≥1000V DC and apply anti-fouling coating.
· Process control: Use automated welding/spraying equipment and set up a clean workshop.
· Environmental management: Install temperature and humidity monitoring and pre-dry materials before construction.
· Test specifications: Segmented testing (500V pre-test + 1000V formal test), calibrate instruments and record data.
(2) Module installation stage
a. Analysis of potential failures and causes
· Assembly error:
Cause: The insulation liner is missing and the bolts are too tight and the insulation layer is damaged.
Performance: Low insulation resistance between the module and the shell, and withstand voltage breakdown.
· Mechanical damage
Cause: Scratches on handling tools, metal debris piercing the insulation film.
Performance: Local short circuit or sudden failure during operation.
· Design compatibility
Cause: Insufficient electrical clearance due to size deviation between the module and the tray.
Performance: Parasitic capacitance causes voltage superposition breakdown.
· Electrical connection hazards:
Cause: Wear and failure of connector seal due to unfixed wiring harness.
Performance: Wear and leakage of high-voltage wiring harness insulation layer.
b. Countermeasures
· Error-proof design: Use positioning pin tooling to prevent missing installation, and customize bolt length to prevent over-tightening.
· Clean operation: Vacuum cleaning before installation, and wrap tools with anti-scratch silicone.
· Tolerance simulation: Verify the matching degree between the module and the tray through CAE to ensure that the gap is ≥10mm.
· Process inspection: After installation, perform random inspection with a megohmmeter (≥100MΩ) and perform segmented withstand voltage test to locate the fault point.
(3) System Integration Phase
a. Potential Failure and Cause Analysis
· Cross-system interface failure:
Cause: Component insulation parameters do not match (such as connector withstand voltage rating differences).
Performance: High-voltage busbar connection breakdown or BMS interference.
· Parasitic capacitance superposition:
Cause: The total parasitic capacitance increases after multiple modules are connected in parallel, and the capacitance rise effect is significant.
Performance: System-level test leakage current exceeds the standard.
· Environmental stress failure:
Cause: Vibration causes insulation fatigue and coolant seeps into the high-voltage interface.
Performance: Insulation resistance decreases periodically during operation.
· Test blind spot:
Cause: Failure to simulate real working conditions (high temperature/vibration/humidity).
Performance: Passed in the laboratory but failed in actual operation.
b. Countermeasures
· Compatibility design: Unify supplier component standards.
· Parasitic capacitance control: Add polyimide isolation layer and optimize layout through SPICE simulation.
· Working condition simulation test: perform "-40℃~85℃ cycle + 5Hz~200Hz vibration + withstand voltage" composite test.
· Intelligent monitoring: integrated online insulation monitoring module, AI predicts potential risks.
3-Insulation withstand voltage test
Insulation withstand voltage test is both a safety measure and a possible cause of failure. The insulation withstand voltage test itself may cause failure due to improper operation, equipment problems or design defects, and even cause secondary damage to the device under test.
(1) Common types of failure during the test
(2) The response strategies are as follows:
a. Precise control of test parameters
· Voltage and time: set according to standards; enable dynamic compensation algorithm for capacitive loads (such as battery modules) to suppress the capacity increase effect.
· Leakage current threshold: set according to equipment specifications (such as ≤10mA) and distinguish between DC/AC modes (DC testing requires a lower threshold).
b. Equipment and operation specifications
· Instrument calibration: The withstand voltage tester is calibrated every 6 months, and the high-voltage line insulation layer is visually inspected daily.
· Safe operation: forced disconnection of low-voltage circuits before testing; use robotic arms or automatic fixtures to avoid manual contact with high-voltage areas.
c. Failure prevention and repair
· Segmented testing: test complex systems in modules (such as testing the high-voltage bus first, then testing the module insulation) to locate the breakdown point.
· Failure repair: use epoxy resin to fill the damaged area after breakdown, and after repair, it is necessary to pass the full voltage gradient test again (such as 500V→1000V step pressure).
d. Environment and data management
· Environmental control: Start the dehumidifier when the humidity in the test area is greater than 75%, or delay the test until the environment meets the standard.
· Data traceability: Record the test voltage, leakage current curve, and environmental parameters, and use the MES system to associate the production batch number.
We will regularly update you on technologies and information related to thermal design and lightweighting, sharing them for your reference. Thank you for your attention to Walmate.
The battery tray uses insulating materials mainly to prevent current leakage, protect personnel safety, and ensure the normal operation of the battery system. When selecting, it is necessary to consider the material's insulation properties, heat resistance, chemical stability and mechanical strength. These factors together determine the application effect of the insulation material in the battery tray, thus affecting the safety and reliability of the entire battery system.
Part 2 - Pressure resistance performance guarantee
Figure 1 Electric vehicle battery tray
1-Insulation material selection and solution design
In the selection of insulation materials for battery trays, the focus is on the dielectric properties, environmental tolerance and mechanical properties of the materials. The following are 6 commonly used insulation materials in battery trays and their related information:
When designing a solution, we generally need to comprehensively consider the three key factors of application scenario, performance requirements and cost budget to select the appropriate insulation material. For example:
(1) Select according to the application scenario: In a high-voltage, high-current energy storage battery system, if the battery tray side requires high-performance insulation material, it is recommended to use the PI film attachment solution; if the insulation performance requirements are not high, the insulation powder spraying solution can be selected. For the battery tray bottom plate, if a low-cost insulation solution is sought, the insulation powder spraying or insulation paint coating solution is more suitable.
(2) Select according to performance requirements: When the requirements for insulation performance, high temperature resistance, chemical corrosion resistance, etc. are high, the PI film attachment solution is a better choice; if these performance requirements are relatively low, the insulation powder spraying or insulation paint coating solution can meet the needs.
(3) Select according to the cost budget: When the cost budget is limited, the insulation powder spraying or insulation paint coating solution is more economical; when the cost budget is sufficient, the PI film attachment solution can be selected.
2-Technical requirements for PI film for battery trays
(1) Material: PI, base film thickness 0.1-0.14mm, back adhesive thickness 0.03mm, PI film thermal conductivity > 0.3W/(m·k);
(2) Pressure resistance: AC 3000V, 60S, leakage current ≤ 0.5mA;
(3) (cold paste) 180° peel strength ≥ 15N/24mm;
(4) Insulation: DC 1500V, 60S, insulation resistance > 1000MΩ;
(5) Heat and electrolyte resistance insulation performance: at 500℃, 700V DC voltage, add 2ml electrolyte (test area 13000mm<H>2<H>), keep for 1h, no breakdown and no sparking;
(6) Heat and insulation performance: at 500±2℃, continue baking (muffle furnace) for 0.5h, the overall morphology of the sample changes without spontaneous combustion, and the sample passes twice. AC 1000V is applied to the surface, and the voltage is increased from 0V to 3000 for 10S, and then continued for 60S. The tested sample has no breakdown and no sparking;
(7) Flame retardant grade: UL94 V-0;
(8) High temperature and high humidity: After 1000 hours of 85℃ and 85% humidity test, the sample has no cracking and no deformation, meeting the national standard "GBT 13542.6-2006 Electrical insulation film Part 6: Polyimide film for electrical insulation" on insulation resistance, withstand voltage value, and electrical strength requirements. The tensile strength, elongation at break, and peel strength are reduced by less than 30% compared with the unaged material;
(9) Hot and cold shock: 85℃ for one hour, switched to -40℃ for one hour, and then switched to 85℃ for high and low temperature cycles. The switching time is included in one hour, and the switching time is ≤3min. After 1000 hours of high and low temperature cycling, the sample has no cracking and deformation, meeting the national standard "GBT 13542.6-2006 Electrical insulation film Part 6: Polyimide film for electrical insulation" for insulation resistance, withstand voltage value, and electrical strength requirements. The tensile strength, elongation at break, and peel strength are reduced by less than 30% compared to the unaged material.
(10) Salt spray test: Neutral salt spray test (NSS) test in GB/T 10125 standard, temperature 35℃, humidity ≥85%RH, test solution is (5±0.1%) (mass fraction) NaCl, PH=6.5~7.2, continuous spraying for 72h. After the salt spray test, the sample has no cracking and no deformation, meeting the national standard "GBT 13542.6-2006 Electrical insulation film Part 6: Polyimide film for electrical insulation" for insulation resistance, withstand voltage value, and electrical strength requirements. The tensile strength, elongation at break, and peel strength are reduced by less than 30% compared with the unaged material.
(11) The product complies with RoHS.
Figure 2 PI film for battery tray
3-Insulation withstand voltage test methods and solutions to common problems
(1) Insulation withstand voltage test method
Insulation withstand voltage test is an important means to evaluate the insulation performance of electrical equipment, mainly including DC withstand voltage test and AC withstand voltage test. DC withstand voltage test detects whether the insulation material breaks down within a specified time by applying DC high voltage. The equipment includes DC high voltage generator, voltmeter, etc. The steps are applying voltage, maintaining voltage and reducing voltage. AC withstand voltage test applies AC high voltage. The equipment and steps are similar to DC test. Both methods require preparation before testing to ensure that the equipment is well grounded and the surface condition of the insulation material is closely observed during the test.
(2) Common problems and solutions
Common problems in insulation withstand voltage test include insulation breakdown, excessive leakage current and test equipment failure. Insulation breakdown may be caused by insufficient insulation material performance, internal defects or moisture. Solutions include selecting high-quality materials, strengthening maintenance and improving insulation structure. Excessive leakage current may be caused by reduced insulation resistance, surface contamination or insufficient accuracy of test equipment. Solutions include keeping the insulation material clean and dry and calibrating the test equipment. Test equipment failure may be caused by equipment aging, improper maintenance or improper operation. Solutions include regular maintenance, correct operation and timely repair.
We will regularly update you on technologies and information related to thermal design and lightweighting, sharing them for your reference. Thank you for your attention to Walmate.
With the rapid development of new energy vehicles and energy storage technologies, the safety and reliability of battery systems, as core energy carriers, have attracted much attention. As the supporting and protective structure of the battery module, the electrical insulation performance of the battery tray is directly related to the safe operation of the vehicle, battery life and personal safety of the user. The electrical safety design of the battery tray is the cornerstone of battery system safety. Through multi-level protection, such as insulation, structure, heat, and monitoring, it ensures stable operation of the battery under complex working conditions, reduces the risk of fire, explosion, or electric shock caused by electrical failure, and extends battery life and improves system reliability.
This article focuses on the electrical insulation safety of battery trays, systematically explains its design principles, the verification method of pressure resistance performance, and the root cause analysis and improvement strategies of typical failure cases, in order to provide theoretical support and practical reference for the high-safety design of battery systems.
Previous article - Design points
The core of the electrical safety design of the battery system lies in the trinity of "prevention-control-emergency": prevent failures through insulation isolation, reliable connection, thermal management and other measures; achieve real-time control with the help of sensors and BMS; use fire prevention, pressure relief and other designs to deal with extreme situations. All key points need to work together to ensure the safety and reliability of the battery system throughout its life cycle, while taking into account maintainability and compliance. The battery tray is not only a structural support in the battery system, but also has multiple functions such as electrical isolation, thermal management, anti-slip, mechanical protection, grounding and modular design, which is crucial to ensuring the electrical safety of the battery system.
1-Structural support ensures electrical connection reliability
The battery tray ensures the reliability and safety of electrical connections under complex working conditions by fixing battery modules, reducing mechanical stress, resisting vibration and shock, maintaining alignment, and integrating environmental protection and thermal management.
Figure 1 Battery tray
A.Physical support and fixation ensure the precise alignment of electrical connection points (such as busbars, wiring harnesses, and connectors) to avoid poor contact caused by structural deformation or displacement; provide a rigid frame to fix battery modules and connectors to prevent loosening or breakage caused by vibration or impact.
B.Environmental isolation and protection: prevent the intrusion of water vapor, dust, salt spray, etc. through sealing design (such as IP67/IP68), avoid short circuits caused by corrosion or insulation failure; block external mechanical shock or foreign body impact to protect high-voltage connection components.
C.Thermal management synergy: integrated heat dissipation structure (such as liquid cooling plate, thermal pad) balances temperature to prevent local overheating from causing oxidation or welding of connection points; reduce thermal interference between adjacent modules through thermal insulation design to avoid material expansion differences caused by temperature gradients.
D.Electromagnetic compatibility (EMC) support: suppress electromagnetic interference through metal shielding layer or conductive coating to protect low-voltage signal lines (such as BMS communication lines) from high-voltage circuit interference.
2-Isolation protection builds an efficient isolation environment
The focus of battery electrical isolation design is to create an environment that can effectively isolate high voltage electricity and ensure efficient operation of the system, ensuring that the battery module can be safely packaged under any operating conditions, preventing accidental release of electrical energy, and thus avoiding potential electrical risks.
A.The battery tray structure takes into account both load-bearing and isolation protection:
l Aluminum alloy materials, such as extruded aluminum alloy, are preferred to achieve lightweight while maintaining high rigidity and impact resistance. The outer frame is used to bear the weight of the entire battery system and external impact. Closed-section profiles are used to enhance structural strength. The inner frame is designed to support battery modules and water-cooled plates, etc., to ensure their stability and heat dissipation requirements.
l Insulating materials are used as pads or coatings to ensure good electrical isolation between the battery module and the tray. The high-voltage wiring harness should have a dedicated management path and insulating sheath to ensure electrical clearance and creepage distance with the tray.
l Advanced welding technologies such as stir friction welding are used to improve connection strength while reducing heat-affected zones, avoiding deformation and potential cracks. For parts that are inconvenient to weld, bolt connections or riveting are used, combined with sealants, to ensure the reliability of mechanical connections and electrical isolation.
l Modularity is considered during design to facilitate battery replacement and maintenance without affecting the stability of the overall structure.
Figure 2 Schematic diagram of electrical clearance and creepage distance
B. Key points of high and low voltage isolation design:
l The positive and negative points of the battery system must be isolated from the low-voltage power supply system and the battery tray to ensure that there is sufficient electrical clearance and creepage distance between the high-voltage circuit and the low-voltage control circuit to meet safety standards and prevent high voltage leakage to the low-voltage system.
l The high-low voltage isolation design needs to consider electromagnetic compatibility (EMC) to ensure that the isolation measures will not introduce interference and keep the system running stably.
l High-impedance connection, the high and low systems are connected through high-impedance, and only the vehicle body ground (battery tray) is allowed to limit the flow of current to ensure that the high-voltage system fault spreads to the low-voltage system.
l Physical isolation measures, when designing the battery tray, the high-voltage and low-voltage components can be arranged in different closed chambers to reduce mutual influence through physical separation; use insulating materials as pads between the battery module and the tray, such as polymer plastics or rubber, to ensure physical and electrical isolation.
l Consider the maintainability of the isolation measures during design to ensure that they can be repaired or replaced safely when necessary.
C.Key points of contact protection design:
l High-voltage harness management: High-voltage harnesses should be properly wrapped in insulating sheaths and managed in an orderly manner through fixing clips or wire troughs to avoid exposure and reduce the risk of direct contact.
l Safety partitions: Insulating partitions are set between battery modules and between battery modules and tray walls to prevent the risk of indirect electric shock caused by electrolyte leakage when the battery is damaged.
l High-voltage component packaging: Key components such as high-voltage connectors and relays are packaged to ensure that these components will not be directly touched by accident even inside the tray.
l Enclosed design: The battery tray is designed as a closed structure as a whole, using a metal or composite shell to ensure that the internal high-voltage components are not exposed, and the shell itself must also have good insulation properties.
l Locking mechanism: For maintainable high-voltage connection points, a locking mechanism is used to ensure that they will not be easily opened during non-professional operations, reducing the risk of accidental contact.
l Insulating material application: Insulating materials are used as an isolation layer between the battery tray and the battery module to ensure that even if the tray is damaged, the human body can be prevented from directly contacting the live parts. These materials include but are not limited to polymer plastics, rubber gaskets or coatings.
3-Electrical Logo Design
The electrical identification of the battery tray can not only improve the safety of operation, but also simplify the maintenance process and reduce the risk of incorrect operation.
A.Clear identification
l Clearly mark warning signs such as "High Voltage Danger" and "Do Not Touch" on the battery tray and surrounding high-voltage components to ensure quick identification even in an emergency.
l Use internationally accepted color coding, such as red or orange to mark high-voltage areas and blue for direct current, to intuitively distinguish different electrical characteristics.
l Apply standardized electrical safety symbols, such as the graphic symbols in IEC 60417, to indicate high voltage, grounding, power-off points, etc. to ensure global understandability of information.
l Include the serial number, production date and batch information of the battery tray for easy tracking and recall management.
l Select wear-resistant and corrosion-resistant materials and printing technologies to ensure that the label remains clear and readable throughout the life cycle of the battery tray.
B.Warning signs
l High voltage hazards are clearly marked on the battery tray and surrounding areas to remind maintenance personnel to pay attention to electrical safety and comply with operating procedures.
l Indicate safe operating distances, especially near high-voltage connectors and exposed locations, to remind people to keep an appropriate distance.
C.Operation guide
l Clearly mark the grounding locations of the battery system and the tray to ensure proper implementation of grounding measures.
l Identify safe test points and maintenance access points, which should be designed to operate at low voltage or non-powered conditions.
l Basic operating and safety instructions can be briefly listed in non-critical areas of the tray to guide the correct operating process.
We will regularly update you on technologies and information related to thermal design and lightweighting, sharing them for your reference. Thank you for your attention to Walmate.
The most popular water blocks are basically pure copper microchannel type. The copper bottom plate is directly processed with skived, and the fins are integrated with the bottom plate to reduce thermal resistance. The bottom plate and the cover plate are brazed or diffused to ensure the reliability of the seal.
1- Advantages of using skiving process to manufacture water blocks
l Integrated design: The skiving process can integrate the floor and fins, which is helpful to reduce contact thermal resistance and improve thermal conductivity. In addition, the integrated bottom plate and fin design can also improve structural strength.
l High-precision processing: The skiving process can produce a very fine tooth structure, and the tooth height, tooth thickness and tooth pitch can be precisely controlled, which makes the heat sink fins denser, the heat dissipation area larger, and the heat dissipation more efficient. At the same time, it can also better meet the personalized needs of different customers for shape, size, etc.
l The production efficiency is high, and the skiving process can be mass-produced. Compared with traditional CNC, the skiving process can process multiple gear pieces at the same time, which greatly improves the production efficiency.
Figure 1: Water block base with different processing technologies a-Skiving b-CNC c-Cold Forging
2- Cost structure of skiving water block
l Design and development costs: The design complexity of the skiving water blockis relatively high, especially when high heat dissipation performance requirements are required, which requires complex process design and optimization.
l Material cost: The materials used in the skiving process are mainly aluminum and copper alloy. The design of combining aluminum plate and copper alloy is more common in radiator manufacturing and has a higher cost performance, so the quality of aluminum and copper directly affects the cost.
l Processing cost:
Skiving process cost: The skiving process involves high-precision CNC machining equipment, such as three-axis CNC machine tools. The use of such equipment requires a high investment cost, and the technical requirements for operators are also high, which will increase production costs.
Welding process cost: In the manufacturing process of water blocks, welding process is also an important cost factor. Vacuum brazing and diffusion welding are two commonly used welding methods. Brazing can weld multiple joints at the same time, has high production efficiency, requires brazing materials, has high requirements for process conditions, and is difficult to control quality; diffusion welding equipment has a large one-time investment, and diffusion welding does not require fillers, but has high requirements for the surface processing of the workpiece.
Surface treatment cost: Common surface treatment methods include anodizing, plating, etc. For aluminum alloy water blocks, anodizing can improve surface hardness, wear resistance and corrosion resistance, while increasing the aesthetics of the product; copper water blocks will be plated, such as nickel plating, to prevent copper oxidation and corrosion, and increase product life and reliability.
l Other costs: inspection and testing, packaging and transportation, etc.
3-Cost optimization suggestions
l Optimizing material usage
Choose the right material: For example, copper has high thermal conductivity, high unit density, and high unit price; aluminum has light weight, low unit price, and slightly poor thermal conductivity.
Material usage: Ensure efficient use of materials and reduce material waste by accurately calculating material thickness and margin.
Figure 2: Schematic diagram of material consumption calculation
l Optimizing production processes
Simplify molds: Design simple molds to reduce mold complexity and cost.
Reduce scrap rate: Reduce scrap rate through precise process control and quality inspection.
One-step molding: Optimize process routes, reduce multiple processing steps, and improve production efficiency.
We will regularly update you on technologies and information related to thermal design and lightweighting, sharing them for your reference.Thank you for your attention to Walmate.
As a key component of the liquid cooling system, the design of the water block must take into account multiple factors such as heat exchange performance, structural strength, corrosion resistance, leakage resistance, and cost control. The water block is usually designed with a complex heat exchange slot structure (i.e., flow channel), and the quality of its internal flow channel design directly determines the heat exchange efficiency of the entire system.
Part 2:Water block design, processing technology and challenges
1-Design requirements for water blocks in different scenarios
l High Performance Computing:
High-performance computing devices (such as high-performance CPUs, GPUs, etc.) generate a lot of heat during operation, so the water block needs to have efficient heat dissipation capabilities. In order to meet the high heat flux density heat dissipation requirements, the water block usually adopts a high-density microchannel design to increase the heat exchange area and improve the heat dissipation efficiency. In addition, some designs integrate the water block directly onto the CPU, eliminating the step of applying silicone grease, which not only simplifies the assembly process, but also further improves the heat dissipation performance. In terms of reliability, the water block must have excellent sealing performance to prevent leakage and ensure long-term stable operation.
l Graphics card cooling:
Graphics cards are high heat generating areas, so the water block needs to have a full coverage design to ensure that all heat generating components on the graphics card can be effectively cooled. At the same time, graphics card cooling requires a high flow rate of coolant, so the internal structure of the water block needs to support high flow rate to quickly remove heat.
l Data Center:
In data centers, the structural design of water block needs to meet multiple requirements such as efficient heat dissipation, low noise, high reliability, adaptability to high power density, intelligent management and environmental adaptability to ensure stable operation and efficient heat dissipation of data centers.
2- Evolution trend of water block structure
The evolution trend of water block structural design reflects the dual pursuit of technological innovation and performance improvement, which is mainly reflected in the following aspects:
l Improved heat dissipation performance:
Increase contact area: Some water block designs improve heat dissipation performance by increasing the contact area with the heating element. For example, a large-area copper base design can achieve good contact and heat conduction.
Optimize the internal structure: Optimize the internal water channel. One idea is to optimize the fluid flow, such as changing from ordinary fins to steering fins, presenting a long strip flow channel, promoting flow boundary layer separation, reducing boundary layer thickness, and improving heat exchange efficiency; another idea is to increase the heat capacity area, such as changing from traditional coarse water channels to microchannel design, which significantly increases the contact area between the coolant and the base plate and enhances the heat dissipation efficiency. In some designs, the coolant is sprayed onto the microchannel base plate through a guide plate to increase the local flow velocity and turbulence, greatly improving the heat absorption efficiency.
l Integrated and intelligent design:
Integrated design: The integrated design integrates the water pump, heat sink fins, heat conduction base and other components together to reduce the number of connection points and improve system stability and heat dissipation efficiency.
Multifunctional integration: In addition to heat dissipation performance, modern water blocks also have temperature display and monitoring functions.
Modular design: The modular buckle structure improves the convenience and freedom of organization.
Figure 1: Water block base with different fin thicknesses
l High-performance materials and finishes:
The use of high-performance materials such as pure copper base, combined with surface treatment technologies such as nickel plating, improves thermal conductivity and corrosion resistance.
3-Processing technology and challenges
l Material properties affect processing:
Material hardness and toughness issues: Radiators made of different materials, such as copper, aluminum and their alloys, have different hardness and toughness, and different requirements for processing tools and processes. Materials with higher hardness will wear faster and require more frequent tool changes; materials with better toughness are prone to deformation and burrs during cutting.
The processing of copper-aluminum composite materials is complex: copper-aluminum composite material shovel-tooth heat sinks must first be made into composite materials using continuous casting semi-molten state pressing technology, and then shovel-tooth processing is performed. The process is more complicated and requires higher precision for equipment and processes.
l High dimensional accuracy requirements
It is difficult to ensure the consistency of tooth height and thickness: For some high-density tooth heat sinks, the height and thickness of each tooth are required to be highly consistent to ensure the performance and uniformity of the heat sink. If the difference in tooth height and tooth thickness is too large, it will lead to uneven heat transfer and affect the heat dissipation effect. During the processing, high-precision equipment and automated control systems are required to ensure that the specifications of each tooth are consistent.
It is difficult to control the tooth spacing: When the teeth on the heat sink are too dense, their density and spacing make the processing process more complicated, and the processing equipment needs to have higher speed and precision to maintain the uniformity of the teeth. For example, when the tooth spacing is too small, the tool is prone to interference during cutting, affecting the processing accuracy and surface quality.
l Strict surface quality requirements
Burr problem: Burrs are easily generated during the processing, which not only affects the aesthetics of the radiator, but also may hinder air flow and reduce the heat dissipation effect. The generation of burrs may be caused by low material cutting accuracy, wear of processing tools, etc., and corresponding deburring processes need to be adopted to solve them.
Surface roughness: The surface roughness of the radiator affects its heat dissipation performance and subsequent surface treatment effects. Excessive surface roughness will increase the resistance to air flow and reduce heat dissipation efficiency. Additional surface treatment is required to reduce the roughness, which increases processing costs and time.
l High processing equipment and process requirements
Equipment accuracy and stability: Gear shoveling requires a high-precision gear shoveling machine, and the accuracy of the equipment directly affects the dimensional accuracy and surface quality of the teeth. At the same time, the equipment must have good stability to ensure dimensional consistency during long-term processing.
Tool selection and wear: Suitable tools are crucial to processing quality. The material, geometric parameters, etc. of the tool need to be selected according to the material properties. During the processing process, tool wear will lead to increased cutting force, decreased dimensional accuracy and increased surface roughness, and the tool needs to be adjusted or replaced in time.
Feed speed and cutting depth: Unreasonable settings of feed speed and cutting depth can easily lead to processing defects. If the feed speed is too fast and the cutting depth is too large, the tool will be overloaded, resulting in tool snatching, tool bounce, tool drop, etc., affecting processing accuracy and surface quality.
Figure 2: Skiving process
l High customization requirements
Different application scenarios have different requirements for the size, shape, tooth height, tooth thickness, tooth spacing and other parameters of the radiator, which need to be customized according to specific needs. This requires the processing manufacturer to have flexible process adjustment capabilities and rich experience to meet diverse customization needs.
We will regularly update you on technologies and information related to thermal design and lightweighting, sharing them for your reference.Thank you for your attention to Walmate.
With the rapid growth of global artificial intelligence computing power and the continuous increase in chip thermal design power consumption (TDP), the cooling demand of data centers is facing unprecedented challenges. At the same time, the increasingly stringent energy-saving and carbon-reduction policies around the world have further promoted the innovation of cooling technology. In this context, traditional air cooling solutions have gradually become difficult to meet the dual requirements of efficient heat dissipation and energy saving. Liquid cooling technology is rapidly rising due to its excellent heat dissipation performance and significant energy-saving advantages, becoming the mainstream choice for data center cooling solutions. As the core equipment for data processing and storage, the performance and stability of the server are directly related to the operating efficiency of the entire system. The core components of the server - including the motherboard, CPU, memory, hard disk and graphics card - will generate a lot of heat under continuous high-load operation. If the heat cannot be dissipated in a timely and effective manner, it will seriously affect the performance and life of the server. To this end, advanced liquid cooling systems have been introduced into server cooling solutions and installed directly on major heat sources such as CPUs and graphics cards to significantly improve heat dissipation efficiency.
Part 1:Water Block Structure and Working Principle
One of the core components of the liquid cooling system is the water block, which is usually made of copper or aluminum materials with high thermal conductivity and is designed with precise water channels and heat sink structures. These water blocks fit tightly to the surface of heat sources such as CPUs and GPUs, and quickly absorb and transfer heat through the cooling water circulating inside. The heat is then transported to the heat sink, which is then circulated through the water cooling system and eventually dissipated into the surrounding air.
Figure 1: Chip thermal power consumption trends of mainstream chip manufacturers
1- Common water block types and characteristics
l Microchannel water block
Features: The microchannel water block adopts a precise micro-water channel design. The water channel structure is fine and complex, which can significantly increase the contact area between the coolant and the heat-generating components, thereby greatly improving the heat dissipation efficiency. The micro-water channel design can also produce a strong turbulence effect during the flow of the coolant, further enhancing the convection heat transfer coefficient and achieving efficient heat transfer.
Application scenarios: It is particularly suitable for CPUs and GPUs with high heat generation, especially in high-performance computing, overclocking, and data centers, which have extremely high heat dissipation requirements.
l Large flow water block
Features: The internal structure of the large-flow water block is relatively simple, usually using a copper plate or etched groove design, and the manufacturing cost is low. Its core advantage is that it relies on high-speed water flow to quickly remove heat, and is suitable for use with a large-flow water cooling system. Although the structure is simple, its efficient heat dissipation capacity makes it a very cost-effective choice.
Application scenario: Suitable for scenarios with certain requirements for heat dissipation efficiency but limited budget, such as mid-to-high-end DIY computer systems or small and medium-sized server clusters.
l Injection type water block
Features: The jet-type water-cooling block sprays the coolant from a narrow nozzle to the micro-channel bottom plate at high speed through the guide plate, forming a strong turbulence effect and significantly improving the heat dissipation efficiency. This design not only increases the contact area between the coolant and the base, but also further optimizes the heat exchange performance through high-speed flow.
Application scenarios: Suitable for high-performance CPUs and GPUs, especially in high-heat dissipation requirements and high-flow scenarios, such as overclocking computing, artificial intelligence training, and graphics rendering.
2-General structure of water block
A water block is a metal block with water channels inside, usually made of copper or aluminum. It does not come into contact with the CPU, graphics card or other heat generating devices. Its structural design directly determines the quality of heat dissipation performance. A typical water block usually consists of the following key parts:
l The base is usually made of high thermal conductivity materials such as copper or aluminum alloy, and the surface is finely processed to ensure close contact with the heat-generating components. The base is equipped with a complex flow channel design to increase the contact area between the coolant and the base.
l The cover plate, together with the base, forms a flow channel sealing cavity to protect the flow channel, seal and other components inside the water block from dust, impurities and external physical damage.
l The water inlet and outlet are the interfaces for the coolant to enter and exit the water cooling head. They are generally designed on the side or top of the water cooling head to ensure that the coolant can flow in and out smoothly. The position design needs to consider the flow path of the fluid to reduce flow resistance and increase the flow rate of the coolant.
l Clips are used to firmly fix the water cooling head to the CPU or other heat-generating components to ensure a tight foundation and achieve efficient heat conduction.
Figure 2: Typical structure of water block
3- Comprehensive optimization of heat dissipation performance
l Flow channel design optimization
Increase the contact area of the flow channel: By designing narrower and denser flow channels, the contact area between the coolant and the base can be significantly increased, thereby improving the convective heat transfer coefficient. For example, the microchannel design has a fine water channel structure. The design parameters of the microchannel water block (such as channel width, height, and spacing) have a decisive influence on its heat dissipation efficiency: as the channel width decreases, the heat transfer coefficient increases significantly, thanks to the enhanced turbulence effect of the coolant flow in the narrow channel, thereby improving the heat exchange efficiency; the higher channel height helps to increase the flow space of the coolant, thereby improving the heat transfer performance; the smaller channel spacing can increase the contact area between the coolant and the heat source, thereby improving the heat dissipation efficiency.
It can also be optimized by optimizing the flow channel layout and reducing the flow channel bends: a reasonable flow channel layout can ensure that the coolant evenly covers the surface of the heat-generating components and reduce the local temperature difference. The bends in the flow channel will increase the head loss and internal flow resistance, thereby reducing the cooling efficiency. The number of bends should be minimized in the design. If it cannot be avoided, the bends should be designed as smooth transitions to reduce the pressure drop and optimize the flow performance.
l High thermal conductivity materials: The base of the water block is usually made of high thermal conductivity materials such as pure copper or aluminum alloy. These materials can efficiently transfer heat from the heat source to the coolant, thereby improving the overall heat dissipation performance.
l Structural parameter optimization, such as base substrate thickness, fins, spoiler structure, etc. The increase in substrate thickness will lead to an increase in the maximum temperature, so the design needs to find the best balance between heat dissipation effect and structural strength; by increasing the height, thickness and spacing of the fins, the heat dissipation performance can be improved, but at the same time, the flow resistance will also increase. Find the best fin design combination to maximize the heat dissipation performance. The shape of the spoiler column can effectively enhance the turbulence effect and improve the heat exchange efficiency.
We will regularly update you on technologies and information related to thermal design and lightweighting, sharing them for your reference.Thank you for your attention to Walmate.
Based on actual production, this article summarizes the common problems, causes and improvement methods in the stir friction welding process for your reference.
1-Surface defects
(1) Surface grooves
l Problem description: Surface grooves, also known as plowing defects, usually appear on the upper surface of the weld, tending towards the advancing edge of the weld, and are groove-shaped.
l Cause: The thermoplastic metal flow around the weld is insufficient, and the plastic metal of the weld cannot fully fill the instantaneous cavity left by the stirring needle during its movement.
l Improvement method: Increase the shoulder diameter, increase the pressure, and reduce the welding speed.
Figure 1: Grooves
(2)Edge burrs
l Problem description: Flash burrs appear on the outer edge of the weld and are wavy in shape.
l Cause: Improper matching of rotation speed and welding speed, excessive downward pressure.
l Improvement method: Optimize welding parameters and reduce the amount of pressure.
Figure 2: Edge burrs
(3)Surface peeling
l Problem description: Surface peeling or threading appears on the surface of the weld in the form of skin or thread.
l Cause: A large amount of heat generated by metal friction accumulates in the surface metal of the weld, causing the local metal on the surface to reach a molten state.
l Improvement method: Optimize welding parameters, reduce rotation speed, and increase welding speed.
Figure 3: Surface peeling
(4) Back weld nodule
l Problem description: Weld bead forms on the back side of the weld.
l Cause: Improper control of the depth and pressure of the stirring head during welding.
l Improvement method: Optimize the depth and pressure settings of the mixing head.
2-Internal defects
(1) Incomplete penetration
l Problem description: A "crack-like" defect occurs when the weld bottom is not connected or is not completely connected.
l Cause: The stirring needle is not long enough, resulting in insufficient stirring of the material at the bottom of the weld.
l Improvement method: Select the appropriate stirring needle length to ensure that the material on the weld thickness can be fully stirred.
Figure 4: Incomplete penetration
(2) Holes
l Problem description: A "crack-like" defect occurs when the weld bottom is not connected or is not completely connected.
l Cause: During the welding process, due to insufficient friction heat input, insufficient material reaches the plasticized state, resulting in insufficient material flow and incomplete closure of the weld area.
l Improvement methods: Optimize welding parameters, improve stirring needle design, and control welding speed and rotation speed.
Figure 5: Holes
We will regularly update you on technologies and information related to thermal design and lightweighting, sharing them for your reference.
Thank you for your attention to Walmate.
Recently, the editor has noticed that many companies are paying attention to and planning immersion liquid cooling energy storage. After a period of silence, immersion liquid cooling technology has attracted attention again and seems to be becoming popular again. I believe that many peers are also paying attention to this development.
1- Features of immersion liquid cooling technology
Energy storage cells are moving towards 300+Ah, and energy storage systems are moving towards 5MWh+. The larger the cell, the more heat it generates, the more difficult it is to dissipate heat, and the more difficult it is to ensure temperature consistency. In addition, the energy storage system is composed of a large number of stacked cells, and the operating conditions are complex and changeable, which is more likely to cause uneven heat generation and uneven temperature distribution. If the problems of heat dissipation and temperature uniformity are not properly solved, the battery's charging and discharging performance, capacity and life will decline, affecting the performance of the entire system. In addition, safety has always been the "Sword of Damocles" hanging over lithium battery energy storage, and the most common way to improve safety is to move towards the three dimensions of intrinsic safety, active safety, and passive safety.
Immersion liquid cooling is to immerse the battery cell in an insulating, non-toxic, heat-dissipating liquid. The coolant has a higher thermal conductivity and specific heat capacity. This direct contact method can provide extremely high heat transfer efficiency while also improving better temperature uniformity. In addition, in addition to being a temperature control medium, the coolant can also be used as a fire-fighting fluid for energy storage systems, combining temperature control and fire protection, which is also a significant feature of immersion liquid cooling technology. Immersion liquid cooling will undoubtedly have more advantages in the context of an industry that requires higher heat dissipation performance and stronger safety.
Figure 1: Immersed liquid-cooled energy storage battery Pack box
2- Immersed liquid cooling energy storage system solution
As a branch of liquid cooling technology, immersion liquid cooling technology is not the first to be used in the energy storage industry. It was initially used in the field of high-performance computing, and later gradually expanded to data centers, artificial intelligence, cryptocurrency, etc.
The original intention of the design of the immersion liquid cooling energy storage system is to solve the shortcomings of traditional air cooling and indirect liquid cooling in cooling efficiency and battery temperature difference control. The official commissioning of the Southern Power Grid Meizhou Baohu project marks the successful application of immersion liquid cooling, a cutting-edge technology, in the field of new energy storage engineering.
l Cooling method and coolant circulation method
The cooling methods are divided into single-phase and phase change. Single-phase immersion liquid cooling is used, mainly including mineral oil, silicone oil, natural ester, etc. Other schemes use two-phase immersion liquid cooling, mainly represented by hydrofluoroether, and use phase change latent heat to dissipate heat and improve heat dissipation efficiency. According to incomplete statistics, the "single-phase immersion cooling" scheme is the most common among the currently released immersion liquid cooling energy storage systems.
According to the difference in the circulation mode of the coolant, there are three technical routes in single-phase immersion liquid cooling: natural convection, pump drive, and immersion coupled cold plate liquid cooling. Natural convection uses the characteristics of liquid volume expansion and density reduction after heating to achieve the floating of hot coolant and sinking after cooling, thereby completing the circulation heat dissipation; the core of the pump drive system is that the liquid cooling unit drives the coolant to circulate between the liquid cooling pipeline and the battery immersion box to complete the entire circulation heat dissipation process; and in the immersion coupled plate liquid cooling scheme, the battery is immersed in the dielectric fluid, and the cold plate in contact with the dielectric fluid is used to take away the heat, avoiding the use of complex secondary circuits to cool the dielectric fluid.
l Product form and integration solution
The integration solution iteration of the immersion liquid-cooled energy storage system is a process from the whole to the part and then to the details. Each step is optimized and improved on the basis of the previous stage to achieve higher performance and safety.
From the cabin level to the pack level, the system integration technology presents the characteristics of scene customization. The diversification of energy storage scenarios makes the demand for energy storage systems different. A single product cannot meet market demand. The modular design allows energy storage products to be optimized and expanded according to the scale and power demand of the project, allowing energy storage solutions to be quickly adjusted and deployed according to different application scenarios and needs.
3-Challenges and implementation scenarios in the industrialization process
Immersed liquid-cooled energy storage systems face many challenges during the commercialization process, including economic feasibility, technical complexity, market acceptance, and industry chain maturity.
l Technical complexity: Compared with cold plate liquid cooling systems, immersion liquid cooling systems are more complex to design and implement.
l Industry chain maturity: The industry chain of immersion liquid cooling technology is not yet fully mature, which limits its application in a wider range of fields. The maturity of the industry chain directly affects the promotion and commercialization of the technology.
l Economic challenges: The energy storage industry is still in the early stages of commercial development, and the lack of profitability makes it difficult for high-cost technology routes to be favored by the market. Many companies compete with low prices for temporary orders, which limits the penetration of immersion liquid cooling.
At present, the main market of the energy storage industry is still dominated by air cooling and cold plate liquid cooling, and immersion liquid cooling has not yet been fully accepted by the market. Although the market penetration and acceptance of immersion liquid cooling technology are not high, it may not show considerable potential in some special scenarios, such as:
l Hazardous chemicals industry: Hazardous chemicals companies have extremely strict safety controls on energy storage equipment, because most of the chemicals they produce and store are highly flammable, explosive, toxic or corrosive. Once an accident occurs, it will not only cause serious losses to the company itself, but may also cause environmental pollution and harm to surrounding communities.
l Base stations and data centers: Base stations and data centers have a low tolerance for thermal runaway. Data center energy storage systems must have batteries with stable performance and are not prone to thermal runaway to ensure system safety. The requirements for power quality are high, and the energy storage system needs to have a fast response capability. In the event of an emergency such as a grid failure or power outage, the energy storage system must be able to directly switch to discharge mode to ensure the continuity and stability of power.
l Fast charging station: When charging and discharging at high rates, the battery generates a large amount of heat in a short period of time, which will cause the battery temperature to be too high and uneven, posing a threat to the battery's performance, life and safety. This means that battery thermal management becomes particularly important in high-rate charging and discharging scenarios.
We will regularly update you on technologies and information related to thermal design and lightweighting, sharing them for your reference.Thank you for your attention to Walmate.
The air tightness of the battery pack is a crucial indicator in electric vehicles and energy storage systems. The air tightness test of the battery pack is mainly carried out on the battery pack shell, interface, connector, cooling assembly, etc. to ensure that the inside of the battery pack is not contaminated or invaded by impurities such as dust and moisture from the external environment, and that the cooling assembly does not leak, so as to ensure that the battery pack maintains normal performance and life, and does not cause safety accidents such as short circuit or explosion.
1-Battery pack protection level and airtightness testing standard formulation
International Protection Making (IEC60529), also known as foreign body protection level or IP Code. The IP (Ingress Protection) protection level system is a standard established by the International Electrotechnical Commission (IEC) to classify the protection level of electrical equipment housings against foreign body intrusion and water intrusion. The airtightness level of the battery pack case is usually required to reach IP67 or IP68, which means that the battery pack case needs to be completely protected from dust ingress (dustproof level 6) and can be immersed in water at a certain pressure for a period of time without water ingress to a harmful level (waterproof level 7). More stringent requirements are that the battery pack can be immersed in 1m deep water for 60 minutes without water ingress (waterproof level 8). The IP protection level usually consists of two digits. The larger the number, the higher the protection level, as shown in Figure 1:
Figure 1: IP protection level description
In order to ensure the battery pack meets the IP67 and IP68 requirements, the battery pack needs to be submerged in water. This method is time-consuming, destructive to the power battery pack, and has certain safety risks. It is not suitable as an offline test for power batteries. Therefore, it has become a common practice in the industry to use airtight testing to ensure that the battery pack meets the IP67 and IP68 requirements. The formulation of airtight testing standards needs to consider the relationship between pressure drop value and leakage rate, as well as the relationship between aperture and water leakage. The formulation of airtight testing standards involves a series of steps from theoretical extremes to experimental verification to achieve the conversion from IP level to airtight testing standards. For example, taking IP68 as an example:
Figure 2: Steps for formulating airtight testing standards
2- Selection of airtightness testing methods and analysis of testing difficulties
The design and manufacturing quality of the battery pack are key factors affecting air tightness, including the toughness and strength of the battery box cover, the sealing of the battery pack shell, interfaces and connectors, explosion-proof vents, and the sealing of the electrical connector itself. In addition, there will be some problems that affect air tightness during use, such as thermal expansion and contraction problems, material aging, and vibration and impact. In the production and manufacturing of battery pack shells, we pay more attention to poor air tightness caused by problems such as welding points and joint quality, such as uneven welding points, weak or cracked welds, air gaps, and poor sealing of joint connections.
The air tightness test of the battery pack is mainly divided into the air tightness test of the upper shell, lower shell, and assembly parts. The air tightness test of the upper and lower shells must meet the air tightness leakage requirements after assembly. When selecting the air tightness test method for the battery pack, the characteristics of the battery pack, test accuracy requirements, production efficiency, and cost are generally considered comprehensively.
Battery pack shell testing in engineering is generally divided into process airtightness testing and shipping airtightness testing. In addition, the airtightness testing of the upper and lower shells must meet the airtightness leakage requirements after assembly, which puts forward more stringent requirements on the testing standards. To ensure that the airtightness meets the requirements, the following difficulties must be overcome in actual operation:
l Product structure stability: the quality of welds, including plug welds, faucet welds, beam welds, frame bottom plate welds, frame front and rear cover plate welds, etc. Weld leakage problems are mainly concentrated in the arc starting and arc ending points and defects caused by burn-through; cracking caused by welding deformation stress, such as bottom plate cavity side wall welding, bottom plate cavity material stratification, and inability to withstand welding deformation stress.
l Adaptability and Stability of Airtight Fixtures: The design of the fixtures should closely match the shape and dimensions of the tested components, ensuring that the components can be securely fixed to the fixtures during the testing process, thereby reducing testing errors caused by positional shifts or vibrations. However, in practice, the size and shape of battery packs vary significantly, necessitating the design and manufacture of multiple different testing fixtures, which increases costs and operational complexity. Designing a universal fixture would further complicate the design process.
l Repeatability of airtightness test results: Factors such as air pressure, temperature, and dryness of the test workpiece/fixture will affect the airtightness test results.
l For workpieces with many non-penetrating tiny cracks, due to the influence of factors such as the accuracy of the detection equipment and the detection parameters, the leakage source may not be discovered, resulting in missed detection.
Figure 3: Airtightness testing tooling
3-Combination of battery pack air tightness detection solutions commonly used in engineering
The air tightness test of the battery pack shell process generally includes air tightness test and water immersion test. In the air tightness test, the upper cover of the battery box is sealed, leaving only a connector port as the air inlet. The air tightness of the battery pack is judged by controlling the air pressure and observing whether there is air leakage. The water immersion test is to completely immerse the entire battery box in water and judge its air tightness by checking whether there is water in the box.
Helium leak detection is a technology that uses helium as a tracer gas to detect leaks by detecting the helium concentration at the leak point. When helium enters the inside or outside of the device being tested where there may be a leak, if there is a leak, the helium will quickly enter or escape the system through the leak and be detected by the mass spectrometer. The helium leak detection method has a high detection efficiency, especially in detecting small leaks.
Figure 4: Comparison of leak detection methods
In actual production, multiple detection methods are usually combined to improve detection efficiency and accuracy. For example, the helium leak detection method is suitable for high-precision and small leak detection, while the differential pressure method has the characteristics of high precision and fast response. In addition, although the traditional water detection method has low detection accuracy, it is intuitive and low-cost, and is a convenient way to locate leaks.
We will regularly update you on technologies and information related to thermal design and lightweighting, sharing them for your reference.Thank you for your attention to Walmate.
The potential failure of the liquid-tightness of the energy storage liquid cooling pack involves multiple aspects, such as: leakage, corrosion and deposition, condensation water and other failure modes.
1- Fluid interconnection and composition
In the energy storage liquid cooling system, the fluid interconnection is responsible for transferring the coolant between the various components. Through effective fluid interconnection, the coolant is ensured to circulate efficiently in the system, thereby removing the excess heat generated during the battery charging and discharging process.
A well-sealed system can effectively prevent coolant leakage. Leakage will not only lead to coolant loss and require frequent replenishment, but also affect the heat dissipation performance and stability of the system. In energy storage, coolant leakage may also cause battery Short circuit, causing safety problems.
2-Liquid-tight design of fluid interconnection system
The liquid-tight design of the fluid interconnection system is the key link to ensure that the system maintains sealing and prevents fluid leakage under various operating conditions.
Figure 1: Typical deployment of energy storage liquid cooling system
(1) Analyze possible leakage sources and risk points in the system:
l The self-sealing property of the liquid cooling assembly. For example, in the integrated design of the liquid cooling channel system and the Pack box, the components are connected by welding. Welding quality defects, poor welding, pores, cracks, etc. may all lead to liquid seepage problems.
l The structural design is unreasonable. For example, the positioning holes or threaded holes of the liquid cooling box are too close to the flow channel, and the poorly welded parts can easily become channels for liquid seepage.
l Connection parts: The pipe connections, valves and joints of the liquid cooling system are common leakage points. If the connection structure is not designed properly or the manufacturing process is not sophisticated, there are tiny defects inside the joints, and the coolant may also leak from these defects.
l Leakage caused by improper installation, material aging or damage, etc.
(2) Sealing structure design:
l The liquid-cooled PACK uses a dry-wet separated cold plate cooling method. Under normal working conditions, the battery cells have no contact with the coolant, which can ensure the normal operation of the battery cells. One solution for the energy storage liquid cooler is to form it through an extrusion process, integrate the flow channel directly on the cold plate, and then use mechanical processing to open up the cooling circulation path. In this process, choosing the right welding process is an important step to ensure sealing. For details, please refer to "Design of welding process for lower box for energy storage".
l Liquid cooling pipelines are mainly used for transitional soft (hard) pipe connections between liquid cooling sources and equipment, between equipment, and between equipment and pipelines. The main connection methods are:
Quick-connection: One of the connection methods for energy storage liquid cooling systems is to use VDA or CQC quick-connection.
Threaded connection: Both ends of the connection structure are slidably connected with pipes, and the threaded connection between the internal thread ring and the threaded sleeve increases the firmness of the connection.
Limiting pipe and nut connection: A connecting pipe is clamped at one end of the pipe, and limiting pipes are fixedly installed on both sides of the connecting pipe. Rubber washers and convex rings are fixedly installed inside the limiting pipes, and a limiting ring groove is opened on the surface of the connecting pipe head. A nut is rotatably connected to the top of the limiting tube and is rotatably connected to the limiting tube through threads.
Sealing ring connection: A sealing ring is adhered to the inner wall of the threaded sleeve by strong glue, and the inner wall of the sealing ring is movably connected to the outer surface of the pipe to prevent leakage during use.
(3) The PACK liquid cooling plate, cabin interface, cabin pipeline, etc. are all designed with long-term corrosion protection under common coolant, common temperature and flow rate conditions to ensure long-term operation without corrosion. Effect of operating conditions on liquid tightness:
l Temperature. Influence of high temperature: As the temperature increases, the viscosity of the liquid generally decreases, which may cause the sealing performance of the liquid to decrease, thereby affecting the liquid tightness. For example, certain sealing materials may deform or deteriorate at high temperatures, causing leakage. Influence of low temperature: In a low temperature environment, the liquid may become viscous, increasing the difficulty of flow, but it may also improve the performance of the sealing material, thereby enhancing liquid tightness to a certain extent.
l Pressure. High pressure environment: Under high pressure, the density and viscosity of the liquid may increase, thereby improving the sealing performance of the liquid. However, excessive pressure may also damage the sealing material and cause leakage. Low-pressure environment: Under low pressure, the sealing performance of the liquid may be relatively weak, especially if the sealing material itself is defective or aged, it is more likely to leak.
l Flow rate. High flow rate: When the liquid flows at high speed, it may produce a large impact force on the sealing surface, causing wear or deformation of the sealing material, thereby affecting the liquid tightness. Low flow rate: At low flow rate, the sealing performance of the liquid is relatively good, but this may also mask some potential sealing problems such as minor material defects.
3-Corrosion and deposition problems
l Effects of blocking on confidentiality:
Coolant, deposits or boiler growth may cause internal blockages, poor coolant flow and reduced cooling efficiency.
Fouling and scaling: Minerals in the coolant may form deposits on the inner wall of the pipe after long-term operation, which is called "scale". Fouling may also be formed due to solid particle precipitation, crystallization, corrosion or microbial activity. These dirt will clog pipes and cold plates, increase flow resistance and reduce heat transfer efficiency.
Foam problem: Foam may be generated in the liquid cooling system. The foam will adhere to the surface of the cold plate, resulting in a decrease in heat transfer effect, and may increase the resistance in system operation, cause cavitation corrosion to the pump, etc., and damage the equipment.
l The influence of eddy current on air tightness:
When a fluid flows in a pipe or gap, changes in velocity can cause eddies to form, especially when the fluid passes through narrow parts or obstacles, eddies are more likely to form. The viscosity and density of the fluid also affect the generation of vortices. Fluids with higher viscosity are more likely to form vortices, while fluids with higher density may weaken the formation of vortices.
Leakage paths: Eddy currents form vortices on contact surfaces, which may form tiny leakage paths in gaps or irregular surfaces, resulting in leakage of gas or liquid.
Surface wear: Eddy flow can cause wear of contact surfaces, especially in high-speed flow conditions. This wear can further reduce air tightness because the worn surfaces are more likely to form new leakage channels.
Thermal effects: Eddy current flow generates heat, which may cause deformation or thermal expansion of the contact surface material, thus affecting the airtightness, especially in systems with large temperature changes.
4-Condensation water problem
Under certain conditions, condensation may form in the liquid cooling lines, which may cause damage to the equipment or reduce efficiency. Insulation failure: If the insulation material of the pipe is damaged or aged, heat will be lost and the cooling effect will be affected. Especially in low temperature environments, insulation failure can cause frost or ice to form on the pipe surface. Frost cracking: In cold environments, if proper antifreeze measures are not taken, the coolant in the pipes may freeze and cause the pipes to rupture.
Solutions
l Sealing measures: Ensure that the inlet and outlet of the liquid cooling pipeline are completely blocked to prevent external humid air from entering the battery compartment.
l Dehumidification equipment: Install a dehumidification air conditioner or use the dehumidification function to maintain the humidity in the battery compartment within an appropriate range.
l Temperature control: By installing air conditioning or ventilation systems, the temperature and humidity of the environment where the energy storage cabinet is located can be controlled. For example, the temperature can be kept at 20-25 degrees Celsius and the relative humidity can be controlled at 40%-60%.
l Isolation measures: Simple isolation of empty battery racks to prevent moisture from entering the compartment containing the battery cluster.
We will regularly update you on technologies and information related to thermal design and lightweighting, sharing them for your reference. Thank you for your attention to Walmate.
The fully sealed design of the energy storage pack is the key to ensuring its safety and long-term stable operation. Sealing is essentially the use of a device to close (seal) a gap or make a joint leak-proof. The fully sealed design can effectively prevent liquid and gas leakage within the battery cell, which is crucial to ensuring the safe and stable operation of the energy storage system. Therefore, when designing, both air tightness and the sealing of the liquid medium must be considered.
In actual operation, the energy storage pack sealing design needs to comprehensively consider multiple factors such as materials, processes, testing equipment, environmental conditions, and manufacturing processes to ensure that its sealing performance can meet the expected standards. This article explains the application practice and key points of energy storage Pack sealing design in actual engineering from the aspects of Pack box airtightness, liquid cooling cycle liquid tightness and liquid cooling medium.
Previous article: Energy storage pack box airtightness design
The sealing design helps maintain the temperature and pressure inside the energy storage pack stable, which plays a key role in the normal operation and performance of the battery; and the sealing design can reduce the impact of the external environment on the internal battery, such as moisture, dust and other pollutants, etc., thereby improving the reliability and service life of the system. In addition, the use of appropriate sealing materials and structures can effectively improve the wear resistance and aging resistance of seals, enhance the durability of the entire energy storage system, and reduce maintenance costs.
The general idea of airtight design is to analyze the box structure to find out the key areas where leakage may exist, and then take targeted measures according to the specific performance and functional requirements of different areas.
1-Box structure analysis
The box is not only the physical carrier of battery modules and electrical components, but also an important guarantee for the safe and reliable operation of the entire energy storage system. It is the "skeleton" of the energy storage pack, which is generally composed of an upper cover, a lower box, support components, and a sealing Parts and bolts, etc.
Figure 1: Schematic diagram of the energy storage pack box and key areas of focus in the sealing design (e.g., marked with red arrows)
As shown in the figure above, find out where potential leaks may occur:
l Multiple parts connection points, such as: the assembly interface between the upper cover and the lower box, the installation interface between the high and low voltage connectors and the box, the installation interface between the exposed components and the battery box, etc.
l If bolts are used for connection, there may also be a risk of leakage at the installation and fixing point, such as the electrical interface and the front panel installation interface of the box.
l There must be no holes or gaps in the upper cover and lower body of the box to ensure the sealing and protective performance of the box.
Figure 2: Immersion liquid cooling lower box (sheet metal frame + aluminum liquid cooling bottom plate)
2- Sealing design of the installation interface between the upper cover and the lower box
The upper cover can generally be divided into two types: flat type and special-shaped type. Their structural characteristics are also different. For example, SMC composite material, aluminum, no matter what material, in order to reduce the complexity of the self-sealing structure, the upper cover of the battery shell The cover is usually of one-piece design. In addition, the opening requirements of the upper cover should also meet the requirements of the interface, and should be independent of the sealing interface to reduce the impact on the battery pack sealing. The upper cover seal design generally follows the following principles:
l The integrated parts design is adopted to avoid the design of separate parts, thus ensuring the stability of the "self-sealing" performance of the upper cover.
l The positioning holes and positioning features are designed on the edge of the upper cover (outside the sealing interface between the upper cover and the lower tray).
l The sealing interface between the upper cover and the lower box body requires the matching surface to meet the "uniform" and "continuous" sealing requirements.
At present, the mainstream solutions for the lower box of the energy storage pack are: sheet metal box + liquid cooling plate, die-cast box + liquid cooling plate, profile integrated box, die-cast integrated box, etc. Among them, the profile integrated box and other solutions In contrast, it has the advantages of good flow channel bearing capacity and low mold opening cost, and is widely used. The choice of welding process has a great influence on the sealing performance. For weldments of different materials and thicknesses, choosing a suitable welding method can effectively improve the weld quality to ensure the overall strength and sealing performance of the system.
In addition, the sealing design of the lower box should follow the following sealing principles:
l Closed section profiles are used for frame construction, and self-sealing linear connection technology, such as CMT welding technology, is used at the joints.
l Battery trays made of aluminum profiles need to be designed with one or more continuous layers of sealing colloid.
l In the case of an integrated liquid cooling plate in the lower box, it is necessary to consider using colloid seals or self-sealing linear connection technologies, such as FSW friction stir welding technology.
l The sealing interface between the upper cover and the lower box body needs the matching surface to meet the "uniform" and "continuous" sealing requirements. If necessary, the sealing interface should be machined and polished.
Figure 3: Common sealing forms between the upper cover and the lower box
Usually, the upper cover and lower box of the energy storage Pack box adopt a bent flange and a sealing gasket design, as shown in Figure 2. The upper cover, lower box body and sealing gasket are fully compacted and coupled by fastening bolts to ensure that the Pack box meets the relevant requirements of IP67.
3- Sealing design of electrical and communication interfaces and the front panel installation interface of the lower box
The front panel of the box (as shown in Figure 3) is machined with holes on the extruded profile for installing electrical and communication interfaces to achieve functions such as current transmission, communication interaction, and safety control.
Figure 4: Electrical, communication interface and lower cabinet front panel installation interface
The air tightness of the installation interface between the box and the electrical, communication and other interfaces shall follow the following principles:
l The interface shape is designed to be streamlined to reduce the possibility of gas and liquid accumulation and penetration at the interface.
l Precise alignment avoids gaps caused by misalignment of interfaces during installation.
l Pre-seal the interface before installation and add anti-vibration pads or sealants to enhance the initial sealing effect or reduce sealing failure caused by vibration.
In addition, in terms of the selection of fasteners, high-strength, high-torque fasteners are used, and they are tightened multiple times during the installation process to ensure the tightness of the interface. For example, if a butt-weld nut is used, its characteristic is that it can be directly connected to the wall hole of the connected part (the front panel of the box) for butt welding. This structural design can significantly improve the airtightness of the connection part.
Figure 5: Using butt-welded nuts to increase airtightness
4-Seal selection
Seal design and selection are critical as they directly affect the reliability and service life of the system. The following are key factors to consider when designing and selecting seals for energy storage liquid cooling systems:
l The sealing material must have certain chemical and pressure compatibility, and be able to withstand the system operating temperature range, including high and low temperature environments. The material selection of the seal depends on the use environment and service life requirements. Common sealing materials include rubber, polytetrafluoroethylene (PTFE), nylon, metal, etc.
l Leakage freedom: The seal must be able to adapt to the slight deformation that may occur in the system during operation to ensure good sealing effect under various working conditions. Generally, the deformation of the gasket should be greater than 30% and less than 60%, and the sealing interface pressure should be greater than 30kPa.
We will regularly update you on technologies and information related to thermal design and lightweighting, sharing them for your reference.Thank you for your attention to Walmate.
In pure electric vehicles, the weight of the battery pack accounts for about 30% of the curb weight. The lightweight of the battery pack is of great significance to improving the cruising range of the vehicle. Therefore, research on the high specific energy of battery packs is one of the current main research directions for new energy vehicles, and it is also the main way to achieve lightweight electric vehicles. The lightweighting of power battery packs can be carried out in two directions: improving single cells The energy density of the battery pack is optimized, and the related accessories of the battery pack are optimized.
The development of multi-material lightweight battery packs aims to reduce the weight of the battery pack, increase energy density and cruising range, while ensuring safety and reliability by using a variety of lightweight materials. Among the main components of the battery pack, the battery cell body has the highest mass, followed by the lower box of the pack, the upper cover, and BMS integrated components.
1-Lightweight design of the battery pack cover
The upper cover of the battery box is located above the power battery box and is not affected by the sides of the power battery box and will not affect the quality of the entire battery pack. Its functions mainly include sealing and protection. In order to improve the energy efficiency of the entire vehicle, lightweight materials will also be considered in the design. The use of lightweight materials, such as aluminum alloys and composite materials (SMC, FRP, etc.), can significantly reduce the weight of the battery pack.
In addition, the structural design of the upper cover also needs to consider manufacturing efficiency and mass production requirements. When the structure is very irregular, it may be difficult to use stamping forming or bending and tailor welding. The design of the upper cover also needs to consider the connection and cooperation with other components such as the lower box and sealing structural parts to ensure the structural stability and reliability of the entire battery box.
2-Lightweight design of the battery pack lower shell
Aluminum alloy is an ideal material for battery pack shells because of its low density, high specific strength, good thermal stability, strong corrosion resistance, good thermal conductivity, non-magnetic, easy molding and high recycling value. Commonly used aluminum alloy materials include 6061-T6, 6005A-T6 and 6063-T6. These materials have different yield strengths and tensile strengths and can meet different structural needs.
The battery pack shell is usually composed of an aluminum alloy profile frame and a bottom plate, which is welded using 6 series aluminum alloy extruded profiles. Factors such as the size and complexity of the profile section, wall thickness, etc. need to be considered during design to adapt to different structural and functional requirements. For example, components such as frames, middle partitions, floor panels, beams, etc. may adopt different cross-section designs.
Through reasonable structural design and connection methods, the overall strength of the shell can be effectively ensured, the processing difficulty can be reduced, and the weight of the shell can be reduced:
l Thin-walled: By adopting a thin-walled design and using stiffeners to meet strength requirements, the weight of the material can be effectively reduced.
l Hollowing: Introducing hollow sections into structural design to reduce material density.
l Size optimization: Optimize the size of the battery pack to reduce unnecessary material usage.
l Topology optimization: Reduce the space occupied by materials by optimizing the layout of internal components of the battery pack.
l Integrated modular design: Integrate cooling plates, battery pack lifting lugs and other components into the box to reduce the number and weight of individual components.
3-Manufacturing lightweight technology
l Material shaping
At present, there are three main categories of battery box material forming processes: stamping, aluminum alloy die-casting and aluminum alloy extrusion. The overall process flow of the power battery box includes material molding and connection processes, among which the material molding process is the key process of the power battery box. At present, the upper casing is mainly stamped, and the main processes of the lower casing are extrusion molding and aluminum alloy die-casting.
l Connection technology
The battery box connection process is crucial in new energy vehicle manufacturing and involves a variety of technologies and methods to ensure the structural strength and sealing of the battery box.
Welding is the main connection process in battery box processing and is widely used. Mainly include the following methods:
① Traditional fusion welding: such as TIG (tungsten inert gas welding) and MIG (metal inert gas welding). TIG welding has low speed and high quality. It is suitable for spot welding and complex trajectory welding. It is often used for frame tailor welding and side beam small piece welding. MIG welding has high speed and strong penetration ability, and is suitable for full-circle welding inside the frame bottom plate assembly.
② Friction stir welding: Welding is achieved by generating heat through friction. It has the characteristics of good joint quality and high production efficiency.
③Cold metal transfer technology: It is suitable for thin plate materials. There is no heat input during the welding process, reducing deformation.
④Laser welding: high precision, high speed, suitable for complex structure welding.
⑤ Stud welding and projection welding: used for quick connection of specific parts. Stud welding is fastened by studs and nuts, and projection welding is connected by pressing bumps.
The mechanical connection method mainly solves the problems of easy welding and thread slippage of thin plate materials during welding, including:
① Blind rivet nut: used to connect the sealing surface of the box frame and the inner cavity bottom plate. It has the advantages of high fastening efficiency and low use cost. Suitable for threaded connections between thin plates and other components.
②Wire thread insert: used to strengthen the screw holes of aluminum or other low-strength bodies, improve the load-bearing capacity of the screws and the force distribution of the threads, and is suitable for battery module mounting holes and sealing surface mounting holes. Compared with blind rivet nuts, wire thread inserts are stronger and easier to repair, but are generally not suitable for thin-wall installations.
We will regularly update you on technologies and information related to thermal design and lightweighting, sharing them for your reference.
Thank you for your attention to Walmate.
In order to cope with market demands such as large spans, fast iterations, and rich product lines, while ensuring cost reduction, efficiency improvement, and quality assurance, for the automotive industry, product standardization - vehicle platformization is undoubtedly a good strategy. Through battery platformization, the same battery pack solution can be matched for different models, or battery pack solutions composed of the same type of battery cells and similar structures can be matched. This means that as many parts as possible can be standardized, which can shorten the development cycle, save costs, streamline production lines, and improve production efficiency.
ONE:Battery Platformization
The battery platform solution is conducive to the overall planning of products, cost reduction and optimization of production capacity. According to the battery platform strategy of the vehicle platform, it is necessary to consider the intersection and bandwidth of the requirements of each model of the platform, and use as few batteries and battery solutions as possible to be compatible with as many models as possible. In the architecture development of pure electric projects, it is crucial to reasonably arrange the integrated power battery pack. Specific work elements include power and power performance requirements, collision safety, layout location and space, etc.
1-Spatial size boundaries and battery cell standardization
l Available battery pack locations
At present, the mainstream power battery layout is under the floor, including under the front seats, under the rear seats, in the middle channel and at the footrest. This layout can maximize the available area, help lower the center of gravity of the vehicle, improve the handling stability of the vehicle and optimize the collision force transmission path.
Figure 1: Battery pack layout during the development of electric vehicles
l Evolution of battery pack space layout
Split battery pack: A split battery pack space layout is adopted, such as the JAC Tongyue series. The energy module consists of two battery packs, one placed at the original fuel tank position, and the other placed in the trunk where the spare tire is stored.
In addition, engineers are continuously exploring usable space within the original architecture of fuel vehicles, resulting in battery pack layouts appearing in "工" (gong), "T," and "土" (tu) shapes.
This type of design is a minor modification of a traditional fuel vehicle. The space is very limited, and the volume and weight of the battery pack that can be loaded are very limited, so the capacity is difficult to increase and the cruising range is not high.
Integrated battery pack: This is a new product design concept. The design of the entire vehicle revolves around the core component - the battery pack. The battery pack is modularly designed and laid flat on the vehicle chassis to maximize available space.
l Battery pack installation point layout
Reasonable layout of the battery pack is crucial, and the limiting factors in the design are ground clearance, passability, collision safety, power requirements and many other aspects.
Figure 2: Battery pack size design constraints
The vehicle platform needs to define the category, level and positioning of each vehicle model within the platform, and then determine the size and wheelbase of the vehicle. The vehicle layout decomposes the size envelope of the battery pack in the X, Y and Z directions according to the vehicle space. The battery needs to be arranged within the given envelope of the vehicle to ensure that there is no interference between the various systems of the vehicle. The curb weight index can decompose the system quality requirements of the battery pack.
In terms of battery size, the design of power battery packs cannot avoid rigid reference indicators such as vehicle space and curb weight, which means that there is a threshold for the design of battery cells. Constrained by this threshold, the battery cell size will be concentrated in a certain range, such as: the length of square battery cells ranges from 150-220mm, the width ranges from 20-80mm, and the height ranges around 100mm. The changing trend of battery cell size specifications is the result of the complementary relationship between vehicle platformization and battery standardization.
However, the battery platform strategies, vehicle models and understanding of standardization of various automakers are different, resulting in significant differences in the current product solutions. For example, BYD's standardization strategy is to fully replace the blade battery, the size of which is locked at 960*13.5 (14)*90 (102) mm, and the single cell voltage is 3.2/3.3V.
2- Development of Endurance Boundaries and Battery Capacity Solutions2- Development of Endurance Boundaries and Battery Capacity Solutions
The power battery provides energy for the vehicle to travel: battery capacity, discharge depth, and energy density affect the amount of power available. In order to meet the needs of different models, the difference in power consumption of models has become an important concern. The vehicle's cruising range will be affected by factors such as electric drive, battery, curb weight, wind resistance, mechanical resistance, low-voltage power consumption, and energy recovery. The possibility of sharing battery solutions between models with large differences in power consumption is weak, so it is necessary to develop personalized battery power solutions, including battery size, quality, power, and power performance optimization to meet the requirements of cruising performance.
Under the constraints of the pure electric range of the vehicle manufacturing platform, the net discharge required by the battery will be affected by the power consumption of different models. It is necessary to confirm the power consumption distribution of each model on the platform in order to further convert the power consumption bandwidth into the battery demand distribution, and then determine the battery power plan required by the platform.
3-Power performance boundary
The dynamic performance of the whole vehicle includes the power performance under acceleration conditions, constant speed conditions and power preservation conditions at different SOCs and ambient temperatures. The variables corresponding to the battery are the power-voltage characteristics of the battery at different SOCs and temperatures. The power of the battery corresponds to the power requirement of the vehicle's power system, and the voltage corresponds to the rated voltage requirement of the drive motor.
Generally, the evaluation of battery solutions for the entire vehicle platform starts from the acceleration time of 100 kilometers at normal temperature and high power and its battery indicator decomposition, and gradually extends to the battery indicator decomposition over the entire range and under all operating conditions.
SECOND:Battery box development
1-Battery integration and modularization
Optimize the design of battery modules, improve the integration and modularity of battery packs, reduce inactive components, and increase the energy density of battery packs.
Currently popular battery pack integration technologies include CTP, CTB, CTC and other forms. The shape, material and combination of parts have changed with the advancement of integration technology. The overall direction is integration and integration. By reducing the number of independent parts and using one large part to replace several parts, larger and more functional components are formed.
2-Battery box design
The battery case is the carrier of the power battery system assembly, plays a key role in the safe operation and protection of the product, and directly affects the safety of the entire vehicle. The structural design of the battery case mainly includes the selection of shell materials for the upper shell, lower shell and other components of the battery case, and the selection of manufacturing process solutions. The upper cover of the battery case mainly plays a sealing role and is not subject to much force; the lower case of the battery case is the carrier of the entire power battery system product, and the battery module is mainly arranged in the lower case. Therefore, there must be structural measures such as embedded grooves and baffles inside the battery case to ensure that the battery module is reliably fixed when the vehicle is driving, and there is no movement in the front, back, left, right, up and down directions, so as to avoid impact on the side walls and upper cover and affect the life of the battery case.
Figure 3: Battery lower box solution, a-skin frame, b-FSW welding + frame, c-FSW welding + frame
l Battery pack installation point structure design and connection fixation
The battery pack installation point usually adopts a mounting beam structure, which runs through the front and back, and the front end is connected to the front cabin longitudinal beam to form an effective and coherent closed beam structure. The installation points are reasonably arranged according to the weight distribution of the battery pack. The battery pack and the vehicle are fixed in various ways, including bolt fixing, mechanical fixing + adhesive joint hybrid connection, snap-on connection, etc.
Figure 4: Battery pack layout and installation section
The power battery pack is generally installed on the vehicle through multiple lifting lug structures. In addition to the large weight of the power battery pack itself, the lifting lugs must also withstand the road excitation brought by the vehicle's movement, such as stone roads and deep potholes. Such durable working conditions and misuse conditions place higher requirements on the strength of the lifting lug structure.
Figure 5: Different lifting lug connection solutions: a Welded lifting lug b Aluminum extruded frame lifting lug
l Battery box safety and protection structure
Mechanical strength and protection: The battery box should have sufficient mechanical strength to protect the batteries inside from mechanical shock and impact. The battery box needs to be able to withstand vibration, extrusion, and mechanical shock to ensure the safety of the battery under various conditions.
Collision protection: The design of the battery case must take collision safety into consideration, especially for side collisions and bottom collisions. It is usually made of aluminum or steel and connected to the lower tray through an outer frame to provide structural rigidity and enhance collision energy absorption capabilities. In addition, appropriate collision absorption structures should be designed to prevent deformation of the battery case and damage to the battery cells.
Waterproof, dustproof and corrosion-resistant: The battery box needs to be waterproof and dustproof, and usually uses IP67-level sealing gaskets to ensure airtightness. In addition, anti-corrosion measures should also be considered, such as spraying PVC coating on the outside to enhance corrosion resistance.
Explosion-proof and pressure relief design: When a battery explodes, the energy should be released in a concentrated and directional manner through devices such as balanced explosion-proof valves to avoid entering the customer cabin. In addition, explosion-proof measures (such as partial structural destruction) should be taken to prevent the overall rupture of the equipment.
l Sealing design
The design of the sealing surface between the upper cover and the lower case of the battery box plays an important role in the sealing performance, and its design needs to be designed together with the battery box structure and the sealing ring. The sealing surface should be kept in the same plane as much as possible to avoid too many curved structures. Since the upper cover and the lower case are connected by bolts, a large number of bolts are used, so it is particularly important to ensure the coaxiality of the holes. While arranging the bolt hole positions reasonably, the position dimensions should be as round as possible and arranged symmetrically in the X and Y directions. The selection of the number of connecting bolts needs to be comprehensively considered based on the level of sealing and the amount of disassembly and assembly workload.
Figure 6: Upper and lower box sealing design, 1-battery upper cover 2-sealing gasket 3-battery lower cover 4-metal conduit
l Electrical safety and short circuit protection
Connection reliability: The connectors inside the battery box should have the correct polarity connection to ensure the overcurrent capacity of the battery box and the reliability of the electrical/mechanical connections, including relaxation measures, etc.
Electrical insulation and voltage resistance design: The module design adopts double insulation protection. The battery cell itself has a layer of battery cell blue film and a battery cell top patch to meet the insulation and voltage resistance requirements. Insulation and voltage resistance protection is set between the end/side plate and the battery cell, and between the battery cell and the bottom mounting surface.
l Thermal management design
Battery thermal management development runs through the entire cycle of battery pack system design and development, including the design of battery temperature control, cold plate, piping system, etc. The main goal of battery thermal management system design is to ensure that the battery system operates at a relatively suitable operating temperature through heating or cooling control while considering space layout, design cost, lightweight, etc., while reducing the temperature difference between cells to ensure consistency.
We will regularly update you on technologies and information related to thermal design and lightweighting, sharing them for your reference.Thank you for your attention to Walmate.
The battery pack is the core energy source of new energy vehicles, providing driving power for the entire vehicle. We generally evaluate the advantages and disadvantages of battery pack technology from the dimensions of efficiency (energy density), safety, manufacturing and maintenance costs.
In battery design, the voltage of a single cell is only about 3-4V, while the voltage required by electric vehicles is at least 100V. New cars now even have a voltage of 700V/800V, and the output power is generally 200W, so the battery needs to be boosted. In order to meet the current and voltage requirements of electric vehicles, different cells need to be connected in series or parallel.
The battery pack is composed of battery cells, electronic and electrical systems, thermal management systems, etc., which are enclosed by a battery frame structure - base plate (tray), frame (metal frame), upper cover plate, bolts, etc. How to "package" these components and systems into a whole more efficiently and safely has always been a topic of continuous research and exploration for the entire industry.
Previous article: Innovation and development of battery integration technology
The origin of power battery group technology can be traced back to the 1950s, and originated in the former Soviet Union and some European countries. This technology was originally used as an engineering and manufacturing concept to determine the physical similarities of parts (universal process routes) and establish their efficient production.
The core of Group Technology (GT) is to identify and explore the similarities of related things in production activities, classify similar problems into groups, and seek relatively unified optimal solutions to solve this group of problems to achieve economic benefits. In the field of power batteries, group technology mainly involves the technology of integrating batteries from single cells into battery packs (Packs), including structure, thermal management, electrical connection design and battery management system (BMS) technology.
The earlier grouping technology in the automotive field is MTP (Module To Pack), which means that the cells are first integrated into modules, and then the modules are integrated into Packs. This technology is characterized by detachable and replaceable modules, which have good maintainability, but the grouping efficiency is low. With the development of technology, the grouping technology has undergone a transformation from MTP to CTP (Cell To Pack). CTP technology refers to the technology of directly integrating cells into Packs, eliminating the traditional module structure and improving grouping efficiency and production efficiency. In recent years, the industry is also exploring grouping technologies such as CTC (Cell To Chassis), CTB (Cell To Body & Bracket) and MTB (Module To Body) with higher integration efficiency.
In the field of power batteries and electrochemical energy storage, the main technological advances of lithium batteries come from structural innovation and material innovation. The former is to optimize the structure of "cell-module-battery pack" at the physical level to achieve the goal of both improving the volume energy density of the battery pack and reducing costs; the latter is to explore battery materials at the chemical level to achieve the goal of both improving the performance of single cells and reducing costs. This article focuses on the impact of different structural integration technologies on battery pack manufacturing technology and the direction of innovative development from the perspective of battery pack structural integration. The current key technologies for power battery integration are shown in the figure below:
1-MTP has been eliminated
At the beginning of the current wave of electric vehicle development, many oil-to-electric new energy vehicle models have been launched. They continue the spatial layout and styling design of traditional gasoline vehicles. Engineers have assembled a relatively large battery cell module by connecting a certain number of individual battery cells in series/parallel, and then placed several such battery cell modules into the battery pack, which is the familiar "MTP" battery pack. Since the battery pack needs to be "packaged" more than twice, the number of required components is extremely large, and the battery pack appears as "three layers inside and three layers outside", with too many redundant parts occupying more system volume and weight, resulting in poor volumetric energy density and gravimetric energy density of the "MTP" battery pack. Additionally, since the design of gasoline vehicles did not specifically reserve space for the battery, the battery system can only be "squeezed in wherever it fits", leading to poor product competitiveness and user experience.
Since the launch of new intelligent electric vehicle platforms represented by Tesla, native pure electric vehicles have enabled battery packs to be installed in ideal spatial locations in a more efficient and regular manner, the three-electric systems can be more reasonably laid out, and the vehicle's electronic and electrical architecture and thermal management design can be more efficiently integrated. The vehicle's product strength in terms of energy efficiency, endurance, and intelligence has been greatly enhanced.
2-Integrated Technology 2.0 Era——CTP
The MTP structure battery pack has a significant space utilization problem. The space utilization of the battery cell to the module is 80%, the space utilization of the module to the battery pack is 50%, and the overall space utilization is only 40%. The module hardware cost accounts for about 14% of the total battery cost. This low space utilization structure cannot meet the development requirements of new energy vehicles. Under the framework of the battery cell → module → battery pack → body integration idea, if the vehicle wants to load as much power as possible in the limited chassis space and improve the volume utilization, it is necessary to consider the standardization of each integration step. As the market demand for driving range continues to rise, the volume of a single battery module continues to increase, which indirectly leads to the emergence of the CTP solution.
CTP structure technology was born for the consideration of safety, packaging complexity, cost reduction, etc. Under the premise of ensuring the safety of the battery cell, CTP technology reduces the internal cables and structural parts. Compared with MTP technology, CTP technology has no module structure and directly packages the battery cell into a battery pack before installing it on the vehicle.
There are currently two main ideas. One is to regard the Pack as a complete large module that replaces the structure of multiple small modules inside, represented by CATL; the other is to consider using a module-free solution during design, and design the battery itself as the strength participant, such as BYD's blade battery.
The core point of CTP technology is to cancel the module design. The battery cell is directly combined with the shell, reducing the use of end plates and partitions. The problems that follow are the fixation of the battery pack and thermal management.
In fact, the original product of the CTP battery pack was not a pure module-free design, but a design that merged the original small modules into three large modules and two medium modules, and there were also aluminum end plates at both ends, so in theory it is still MTP, but there are indeed great improvements in structure.
After the introduction of CTP 3.0, CATL presented a more advanced manufacturing method, achieving a completely module-free design. The battery cells have been changed from a vertical orientation along the height to a horizontal position. Additionally, a new cooling solution has been implemented between the battery cells, which not only dissipates heat but also provides support, cushioning, insulation, and temperature control functions. The bottom shell has also been designed with a limiting fixation feature.
Figure 1: Comparison between CATL Kirin Battery CTP2.0 and CTP3.0
3-Integrated Technology 3.0 Era——CTB, CTC
a.CTB technology
CTP technology is a major step forward in battery structure innovation, but it has not made a breakthrough in the battery pack itself. In CTP technology, the battery pack is still an independent component. Compared with CTP's streamlined strategy for battery packs, CTB technology combines the body floor panel and the battery pack cover into one. The flat sealing surface formed by the battery cover, the door sill, and the front and rear beams seals the passenger compartment with sealant, and the bottom is assembled with the body through the installation point. When designing and manufacturing the battery pack, the battery system is integrated with the body as a whole, the sealing and waterproof requirements of the battery itself can be met, and the sealing of the battery and the passenger compartment is relatively simple, and the risks are controllable.
In this way, the original sandwich structure of "battery pack cover-battery cell-tray" is transformed into a sandwich structure of "body underbody integrated battery pack cover-battery cell-tray", reducing the space loss caused by the connection between the body and the battery cover. In this structural mode, the battery pack is not only an energy source, but also participates in the force and transmission of the whole vehicle as a structure.
Figure 2: Schematic diagram of CTB technology structure
b.CTC technology
After adopting the CTC method, the battery pack is no longer an independent assembly, but is integrated into the body of the vehicle, which optimizes the product design and production process, reduces the number of vehicle parts, especially reduces the internal structural parts and connectors of the battery, has the inherent advantage of lightweight, maximizes the space utilization, and provides space for increasing the number of batteries and improving the driving range. Under the condition that the electrochemical system itself remains unchanged, the driving range can be increased by increasing the number of batteries.
Figure 3: Tesla CTC technology structure diagram
For example, Tesla and other automakers have successively launched CTC technology models. At the cell level, they can use multifunctional elastic sandwich structures and large-area water cooling technology, and superimpose the anti-collision space reuse technology at the bottom of the battery pack brought by integrated development, taking into account the grouping efficiency, heat dissipation and safety, and promoting the application of CTC technology from the two dimensions of cell optimization and vehicle structure protection. At the level of vehicle integrated development, the battery cell is directly integrated into the chassis, eliminating the links of modules and battery packs. The integration of the three major electric systems (motor, electronic control, battery), the three minor electric systems (DC/DC, OBC, PDU), the chassis system (transmission system, driving system, steering system, braking system) and autonomous driving related modules is realized, and the power distribution is optimized and energy consumption is reduced through the intelligent power domain controller.
4-Changes in specific requirements for battery boxes for CTP, CTB and CTC technologies
In the traditional battery pack structure, the battery module plays the role of supporting, fixing and protecting the battery cell, while the battery box body mainly bears the external extrusion force. The application of CTP, CTB and CTC technologies puts forward new requirements for battery boxes, which are specifically reflected in:
The strength requirements of the battery box body are improved: Since the module link is reduced or eliminated in the CTP, CTB and CTC structures, the battery box body must not only withstand the external extrusion force, but also the expansion force from the battery cell originally borne by the module. Therefore, the strength requirements of the battery box body are higher.
Collision protection capability: After using CTP technology to remove the side beams of the battery pack, the battery will directly bear the impact of the collision, so the CTP battery pack needs to have sufficient collision resistance.
Insulation, insulation and heat dissipation requirements: CTP or CTB and CTC structures change the bottom plate profile to a water-cooled plate based on the chassis-bearing structural box. The battery box box not only bears the weight of the battery cells, but also provides thermal management and other functions for the battery. The structure is more compact, the manufacturing process is optimized, and the degree of automation is higher.
Reduced maintainability: The highly integrated design makes it complicated to replace the battery pack. For example, in the CTC structure, the battery cells are filled with resin material, which makes it difficult to replace the battery cells and almost impossible to repair.
5- Impact of battery pack integration on electric vehicle charging infrastructure
Choosing different battery pack integration technologies also implies choosing different compensation methods. CTP tends to be battery replacement, while the more highly integrated CTB/CTC tends to be fast charging.
High integration means that more batteries can be accommodated in the same space, thereby increasing the range of electric vehicles. Users may no longer need to charge frequently for short distances, but may prefer to charge quickly during long journeys. Therefore, the planning of charging infrastructure needs to take these changes into account to ensure that it can meet user needs.
As the integration of battery packs increases, the physical size and structure of the battery packs may change, which may affect the design of the charging interface and the compatibility of the charging equipment.
In addition, the increased integration of battery packs may also affect charging speed and efficiency. More efficient battery management systems and charging technologies may need to be developed and deployed to ensure a fast and safe charging process.
We will regularly update you on technologies and information related to thermal design and lightweighting, sharing them for your reference.
Thank you for your attention to Walmate.
In the production process of battery trays and energy storage liquid cold boxes for new energy vehicles, necessary and appropriate surface treatment is a key step, such as: using coating, oxidation treatment, etc. to form a protective layer on the metal surface to resist the erosion of corrosive media; Components that require electrical isolation, such as battery cells, water-cooling plates, module walls, etc., need to establish an insulating protective film. Insulation is generally achieved by spraying insulating powder or insulating paint. Choosing the appropriate surface treatment technology can not only improve the performance of the tray/liquid cooling box Durability and safety can also meet the needs of different application scenarios. This article summarizes common surface treatment technologies for reference.
1-Cleaning and polishing
During the production process, impurities such as processing oil, engine oil residue, powder, and dust may accumulate on the surface of the pallet. Not only do these impurities affect the service life of the battery tray, they may also adversely affect the performance and safety of the battery. Through cleaning and polishing, these impurities can be effectively removed to ensure the cleanliness of the pallet surface. Cleaning and grinding can effectively remove surface impurities, burrs, and welding slag, making the surface smooth and flat, thus improving the overall quality of the battery tray/box.
a.chemical cleaning
Alkali cleaning: Alkaline cleaning mainly uses alkaline solutions (such as sodium hydroxide, sodium carbonate, etc.) to remove grease, dirt and other organic matter on the surface of aluminum alloys. Alkaline washing removes grease through saponification, emulsification and penetration and wetting, and at the same time generates water-soluble precipitates, thereby achieving a cleaning effect. Alkaline cleaning is usually used to remove grease, dust and organic contaminants from the surface of aluminum alloys.
Pickling: Pickling uses acidic solutions (such as nitric acid, hydrochloric acid, etc.) to remove oxide scale, rust and other inorganic deposits on the surface of aluminum alloys. Pickling converts the oxides on the metal surface into soluble salts through the reaction of acid with the oxides on the metal surface, thereby removing surface impurities. Pickling is mainly used to remove oxide film, rust and inorganic salt scale on the surface of aluminum alloys. Pickling is often used for the final treatment of metal surfaces to improve their finish and flatness.
b.Mechanical grinding
During production, the grinding process can remove processing allowances, correct shape errors, ensure the smoothness and accuracy of the pallet/box surface, meet assembly requirements, and thus improve overall performance and service life.
The cleaned and polished surface can be treated with coating materials or other materials, which is very important for the subsequent construction of anti-corrosion, sealing, thermal conductivity, insulation, thermal insulation and other coatings, and plays a key role in the firm attachment of these materials to the pallet/box.
2-Coating and protective film establishment
In addition to basic cleaning and polishing, the production of pallets/boxes uses a spraying process for surface treatment to form a protective layer to prevent oxidation and corrosion and to meet the needs of different scenarios such as thermal insulation, insulation and voltage resistance.
a.Thermal insulation
Anti-condensation and thermal insulation of battery trays can be achieved through comprehensive design of thermal insulation systems, use of high-efficiency thermal insulation materials, application of aerogels, battery pack insulation design, and spraying of foam insulation materials.
Bottom surface sprayed with PVC and foam material
b.Insulation withstand voltage
The insulation of the battery pack casing and the liquid cooling components is primarily to prevent current leakage, protect personnel from electric shocks, and ensure the normal operation of the battery system. Insulation is typically achieved through two main methods: powder spraying and film lamination. The mainstream film lamination processes include room temperature lamination, hot pressing, and UV exposure.
Internal spraying of insulation powder and insulation paint
3-Logos and Signage
A nameplate or label is set in a prominent position on the battery tray, generally through laser, mechanical engraving, etc. These logos are usually made of wear-resistant and corrosion-resistant media to ensure that they are not easily erased during the entire service life.
We will regularly update you on technologies and information related to thermal design and lightweighting, sharing them for your reference.
Thank you for your attention to Walmate.
As the core equipment of the energy storage system, the energy storage converter is an important tool for power conversion, energy management, ensuring grid stability, improving energy efficiency, etc. As the energy storage converter power unit moves toward high integration and high efficiency, the development of frequency and large capacity puts higher and higher requirements on heat dissipation.
1-Changes in cooling requirements
a.Matching the larger DC cabin, the converter capacity continues to increase, and efficient heat dissipation technology ensures the reliability of the equipment.
As the capacity of energy storage cells becomes larger and larger, the capacity of energy storage systems is also expanding simultaneously. At the beginning of 2023, the standard 20-foot single-cell battery capacity on the market was only 3.35MWh. In the second half of the year, many battery cell companies have launched 310+Ah energy storage products, and the capacity of the 20-foot single-cell battery has also been expanded to 5MWh. However, less than half a year after the 5MWh model was updated, some leading energy storage systems released 6MWh and 8MWh systems. According to general experience, the energy storage converter is configured at 1.2 times the load capacity. The single unit capacity of a 5MWh energy storage system must be greater than 2.5MW. High power requires more efficient cooling technology to ensure stable operation of the equipment under sustained high loads.
Iterative evolution of energy storage system integration topology scheme
b.The application of DC high-voltage technology requires devices to have higher withstand voltage levels and insulation strength, and the heat dissipation of power devices is severe.
In order to match the large-capacity energy storage system, DC high-voltage technology has become a technical trend. Through the increase of voltage level, energy saving, efficiency and performance improvement can be achieved. The 1500V voltage upgrade originated from photovoltaics, and now photovoltaics are involved in energy storage. However, the high-voltage evolution of energy storage PCS still has a long way to go, and some manufacturers have begun to optimize and push it to 2000V. The application of DC high-voltage technology forces the power electronic devices in energy storage converters to have higher withstand voltage levels and higher insulation strength to adapt to high-voltage working environments. In high-voltage environments, the heat dissipation design of power devices becomes more important. The pn junction temperature of power devices generally cannot exceed 125°C, and the temperature of the package shell does not exceed 85°C.
c.Networked energy storage systems require complex control algorithms, circuit designs and high power density energy storage converters
Unlike the essential characteristics of current sources in grid-forming energy storage systems, grid-forming energy storage systems are essentially voltage sources that can internally set voltage parameters to output stable voltage and frequency. Therefore, it is required that grid-forming converters simulate the characteristics of synchronous generators, providing support for voltage and frequency to enhance the stability of the power system. This control strategy necessitates that converters possess higher power density and more complex control algorithms, as well as higher-performance power devices and more intricate circuit designs to implement the control strategy. Effectively managing the heat generated by high power density and complex control strategies, while reducing the size and cost of the cooling system without compromising performance, has become a new challenge in thermal design.
2- Comparison of common cooling solutions
The cooling solution for energy storage inverters has undergone significant iterative evolution in recent years, mainly reflected in the transition of cooling technology from traditional air cooling to liquid cooling technology.
a.Air cooling solution
Air cooling is the temperature control form used in the early stage of energy storage converters. It uses air as the medium and dissipates heat through fans and radiators. The air cooling solution improves heat dissipation efficiency by continuously reducing energy consumption, optimizing structure, and improving heat dissipation materials. At the 2.5MW power level, air cooling can still meet the requirements.
b.Liquid Cooling Solution
As the power density and energy density of energy storage systems continue to increase, liquid-cooled PCS uses coolant with high thermal conductivity as the medium. The coolant is driven by a water pump to circulate in the cold plate and is not affected by factors such as altitude and air pressure. The liquid cooling system has a more efficient heat dissipation efficiency than the air cooling system. The liquid cooling solution has a higher matching degree and has begun to be explored and popularized in the past one or two years.
In addition to the full liquid cooling energy storage solution, some manufacturers have launched energy storage direct cooling machines, which use phase change direct cooling and no water circulation. Direct cooling solutions are also entering the energy storage field.
We will regularly update you on technologies and information related to thermal design and lightweighting, sharing them for your reference.
Thank you for your attention to Walmate.
The battery pack is a key component of new energy vehicles, energy storage cabinets and containers. It is an energy source through the shell envelope, providing power for electric vehicles and providing consumption capacity for energy storage cabinets and containers. In combination with actual engineering needs, this article summarizes the key points of profile design for battery packs by analyzing the requirements of mechanical strength, safety, thermal management and lightweight of battery packs.
1-Battery pack housing design requirements
a.Mechanical strength, vibration resistance and impact resistance. After the test, there should be no mechanical damage, deformation or loosening of the fastening, and the locking mechanism should not be damaged.
b.Sealing: The sealing of the battery pack directly affects the working safety of the battery system. It is usually required to reach IP67 protection level to ensure that the battery pack is sealed and waterproof.
c.The design of the battery pack shell needs to take thermal management performance into consideration and ensure that the battery operates within an appropriate range through appropriate thermal management design.
d.For installation and fixation, the shell should have space for nameplate and safety signs, and reserve sufficient space and fixed foundation for the installation of acquisition lines, various sensor elements, etc.
e.e. All connectors, terminals, and electrical contacts of non-polar basic insulation should meet the corresponding protection level requirements when combined.
f.Lightweighting: Lightweighting of the shell is of great significance to improving the energy density of the battery pack. Aluminum alloy is light in weight and high in quality, making it the most feasible choice at present. The lightweighting level can be improved through appropriate extreme design in combination with actual applications.
g.Durability: The design life of the battery pack shell shall not be less than the service life of the overall product. No obvious plastic deformation should occur during the use cycle. The protection level and insulation performance should not be reduced. The structure should be easy to maintain, including the layout of nameplates and safety signs, and protection of connectors.
Figure 1 Typical aluminum alloy welded battery pack shell
2-Typical aluminum alloy battery pack shell solution
Commonly used aluminum alloy materials for battery pack shells include 6061-T6, 6005A-T6 and 6063-T6, etc. These materials have different yield strengths and tensile strengths to meet different structural requirements. The strength of these materials is: 6061-T6>6005A-T6>6063-T6.
At present, the battery pack shell forming solutions include aluminum profile welding, aluminum alloy casting, cast aluminum plus profile aluminum, stamped aluminum plate welding, etc. The aluminum profile welding solution has become the mainstream choice due to its flexibility and processing convenience. As shown in Figure 1, the shell is mainly composed of an aluminum alloy profile frame and an aluminum alloy profile bottom plate, which are welded using 6 series aluminum alloy extruded profiles. The aluminum alloy casting solution is regarded as the future development direction due to its simplified process and cost reduction potential.
3- Profile section design
a. Section size and complexity: The section size of the profile is measured by the circumscribed circle. The larger the circumscribed circle, the greater the extrusion pressure required. The section of the profile is usually composed of multiple cavities to improve the structural rigidity and strength. Usually, the frame, middle partition, bottom plate, beam, etc. adopt different section designs to adapt to different structural and functional requirements.
Figure 2 Typical aluminum alloy profile section
b. Aluminum profile wall thickness: The minimum wall thickness of a specific aluminum profile is related to the profile circumscribed circle radius, shape and alloy composition. For example, when the wall thickness of 6063 aluminum alloy is 1mm, the wall thickness of 6061 aluminum alloy should be about 1.5mm. The extrusion difficulty of the same section is: 6061-T6>6005A-T6>6063-T6. In the design of battery pack profiles, the frame profile is usually made of 6061-T6 aluminum alloy material, and its typical section is composed of multiple cavities, and the thinnest wall thickness is about 2mm; the bottom plate profile is also composed of multiple cavities, and the material is generally 6061-T6, 6065A-T6, and the thinnest wall thickness is also about 2mm; in addition, in the design of the bottom plate load-bearing tray and bottom plate liquid cooling integration, the bottom plate generally adopts a double-sided structure, the bottom plate thickness is generally 10mm, and the wall thickness and the inner wall of the cavity are about 2mm.
c. Tolerance of profile cross-sectional dimensions: The tolerance of cross-sectional dimensions should be determined based on the processing allowance of the aluminum profile, the conditions of use, the difficulty of profile extrusion, and the shape of the profile. For some aluminum profiles that are difficult to extrude, the shape can be changed or the process allowance and dimensional tolerance can be increased to reduce the difficulty of extrusion and extrude aluminum profile products that are close to the requirements, and then they can be reshaped or processed to meet the use requirements.
In addition, when designing the profile section, it is necessary to consider the specific requirements of different welding processes for joints, grooves, wall thickness, etc.
We will regularly update you on technologies and information related to thermal design and lightweighting, sharing them for your reference.
Thank you for your attention to Walmate.
Battery trays, also known as battery boxes or PACK boxes, are increasingly valued as a very important component in the development of new energy vehicles. The design of battery trays needs to balance the relationship between factors such as weight, safety, cost, and material performance. Aluminum alloys are widely used in automotive lightweight engineering because of their low density and high specific strength, which can ensure rigidity while ensuring vehicle body performance.
1-Battery tray welding location and method selection
Aluminum battery trays are made of extruded aluminum profiles, and the various components are combined into a whole by welding to form a complete frame structure. Similar structures are also widely used in energy storage pack boxes.
The welding parts of the battery tray usually include the bottom plate splicing, the connection between the bottom plate and the side, the connection between the side frame, the horizontal and vertical beams, the welding of liquid cooling system components, and the welding of accessories such as brackets and hanging ears. When selecting welding methods, different welding methods will be selected according to different material and structural requirements, see the table below:
2-Analysis of the influence of welding thermal deformation
Welding is a local heating processing method. Since the heat source is concentrated at the weld, the temperature distribution on the weld is uneven, which eventually leads to welding deformation and welding stress inside the welded structure. Welding thermal deformation is the phenomenon that the shape and size of the welded parts change due to uneven heat input and heat output during the welding process. Combined with actual engineering project experience, the parts that are prone to welding thermal deformation and the influencing factors are summarized:
a. Long straight welding area
In actual production, the bottom plate of the battery tray is generally made of 2 to 4 aluminum alloy profiles spliced together by stir friction welding. The welds are long, and there are also long welds between the bottom plate and the side plate, and between the bottom plate and the spacing beam. Long welds are prone to local overheating in the welding area due to concentrated heat input, resulting in thermal deformation.
Battery tray frame welding
b. Multi-component joints
It is caused by local high temperature heating and subsequent cooling during the welding process at the multi-component weld. During the welding process, the weldment is subjected to uneven heat input, resulting in a significant temperature difference between the weld area and the surrounding parent material, which causes thermal expansion and contraction effects, causing deformation of the welded parts. The electrical installation end of the energy storage pack box is usually equipped with a water nozzle, a wiring harness bracket, a beam, etc., and the welds are dense and very easy to deform.
In the weld-intensive area, the front side of the pallet is warped and deformed
c.Cold plate channel side wall
In the battery tray with integrated design of liquid cooling plate, parts with smaller structural rigidity, such as thin plates and pipe structures, cannot resist thermal deformation well during welding and are prone to deformation. For example, the side wall of the liquid cooling plate flow channel is very thin, generally only about 2mm. When welding beams, wiring harness brackets and other parts on the module mounting surface, it is easy to cause cracks and deformation wrinkles on the side wall of the flow channel, affecting the overall performance.
Thermal crack defects on the liquid cooling channel wall caused by beam welding
3-Welding thermal deformation control method
a. Segment welding, double-sided welding
For parts with relatively low strength requirements, segmented welding is adopted, and the welding process is broken down into multiple small sections. The welds are arranged symmetrically, and the welds are arranged symmetrically near the neutral axis in the construction section, so that the deformations caused by the welds may offset each other. At the same time, the length and number of welds are minimized, and excessive concentration or crossing of welds is avoided, which can reduce the welding temperature gradient and thus reduce welding deformation. For parts with high strength requirements such as the bottom plate, bottom plate and side frame, double-sided welding is adopted to increase strength while reducing bending deformation caused by large parts and long welds.
b.Optimizing welding sequence
Control welding deformation, use joints with lower rigidity, avoid two-way and three-way intersecting welds, and avoid high stress areas. Optimize the welding sequence, weld the weaker rigidity areas first, and the better rigidity areas last, such as welding the fillet welds first, then the short welds, and finally the long welds; weld the transverse welds first, then the longitudinal welds. A reasonable welding sequence can effectively control welding deformation, thereby controlling the weld dimensions.
c. Welding parameter adjustment
Control welding parameters and processes, and reasonably set welding speed, number of welding layers and thickness of each weld. For thicker welds, use multi-layer and multi-channel welding methods, and the thickness of each weld layer should not exceed 4mm. Multi-layer welding can reduce structural microstructure and improve joint performance. Accurately control welding parameters and reasonably select parameters such as welding current, voltage, electrode model and welding speed to ensure consistent shape and size of the molten pool, thereby avoiding errors caused by improper parameter selection.
d.Welding skills improvement
Improve the welder's operating skills (use mechanical processing for large components or nodes with strict requirements) to ensure consistency and standardization of actions during welding and reduce dimensional problems caused by human factors.
We will regularly update you on technologies and information related to thermal design and lightweighting, sharing them for your reference.Thank you for your attention to Walmate.
Aluminum alloy is the most widely used non-ferrous metal structural material in industry, especially in scenarios where the thermal conductivity of materials is of great concern, and in situations where efficient heat conduction is required, such as electronic equipment heat dissipation, electric vehicle three-power heat dissipation, and battery energy storage systems. In the fields of heat dissipation and aerospace, it is usually used to manufacture efficient heat transfer equipment such as radiators, heat conduction plates, and electronic components.
Thermal conductivity, also called thermal conductivity, is a parameter index that characterizes the thermal conductivity of materials. It indicates the heat conduction per unit time, unit area, and negative temperature gradient. The unit is W/m·K or W/m·℃. Aluminum alloy is an alloy material composed of aluminum and other metals. Its thermal conductivity is very excellent, and the thermal conductivity coefficient is usually between 140-200W/(m·K). As the metal with the highest content in the earth's crust, aluminum has a relatively low thermal conductivity coefficient. It is favored because of its high height, low density and low price.
1-Thermal conductivity principle of aluminum alloy materials
When there is a temperature difference between adjacent areas of a material, heat will flow from the high-temperature area to the low-temperature area through the contact part, resulting in heat conduction. There are a large number of free electrons in metal materials. Free electrons can move quickly in the metal and can quickly transfer heat. Lattice vibration is another way of metal heat transfer, but it takes a back seat compared to the free electron transfer method.
Comparison of heat conduction methods between metals and non-metals
2-Factors affecting the thermal conductivity of aluminum alloys
a.Alloying is one of the main factors affecting thermal conductivity. Alloying elements exist in the form of solid solution atoms, precipitated phases and intermediate phases. These forms will bring crystal defects, such as vacancies, dislocations and lattice distortion. These defects will increase the probability of electron scattering, resulting in a reduction in the number of free electrons, thereby reducing the Thermal conductivity of alloys. Different alloying elements produce different degrees of lattice distortion on the Al matrix, and have different effects on thermal conductivity. This difference is the result of multiple factors such as the valence of alloy elements, atomic volume differences, extranuclear electron arrangement, and solidification reaction type.
b.Heat treatment is a very important step in the processing of aluminum alloys. By changing the microstructure and phase transformation of aluminum alloys, its thermal conductivity can be significantly affected. Solid solution treatment is to heat the aluminum alloy to a certain temperature to fully dissolve the solute atoms in the matrix, and then quickly cool it to obtain a uniform solid solution. This treatment improves the material's mechanical properties but usually reduces its thermal conductivity. Aging treatment is through appropriate cold deformation and reheating after solid solution treatment, which can optimize the microstructure of the alloy and improve its overall performance. The aging treatment takes into account the mechanical properties and thermal conductivity of the alloy, so that the alloy maintains high strength while also having good thermal conductivity. Annealing improves the microstructure of the alloy by maintaining it at a lower temperature to precipitate and redistribute the second phase in the alloy. Annealing treatment can improve the plasticity and toughness of aluminum alloys, but the effect on thermal conductivity varies depending on the specific situation.
Schematic diagram of crystal structure changes during the aging process of Al-Cu alloy
c.Other factors influence, impurities and second phase particles: Impurities and second phase particles (such as oxides, carbides, etc.) in aluminum alloys can scatter hot carriers (electrons and phonons), thereby reducing thermal conductivity. The higher the impurity content, the coarser the second phase particles and generally the lower the thermal conductivity. The grain size of aluminum alloys also affects thermal conductivity. Generally speaking, the smaller the grain size, the more grain boundaries there are and the lower the thermal conductivity. In addition, the processing method of aluminum alloy (such as rolling, extrusion, forging, etc.) will affect its microstructure and residual stress state, thereby affecting the thermal conductivity. Work hardening and residual stresses reduce thermal conductivity.
In summary, aluminum alloy is an ideal choice for high thermal conductivity materials. Factors such as the type of alloy elements in aluminum alloys and their forms, heat treatment methods, impurities, grain size, and molding methods will all affect the thermal conductivity of aluminum alloy materials. Comprehensive considerations should be taken when designing material composition and process planning.
We will regularly update you on technologies and information related to thermal design and lightweighting, sharing them for your reference.Thank you for your attention to Walmate.
The air tightness of the battery pack is a key factor in ensuring the quality and safety of the battery pack. It is related to the safety, reliability and service life of the battery pack. The air tightness test of the battery pack should be carried out not only during the production process, but also during battery maintenance and inspection.
1-Battery Pack Airtightness Requirements
In actual production, the air tightness of the battery pack must meet the following requirements:
Sealing performance, the battery pack shell, interface and connectors must have good sealing performance to prevent dust, water vapor and other external impurities from entering the battery pack, which can be achieved through welding, sealants, waterproof materials, etc.
Waterproof performance, to prevent moisture from entering the battery, causing short circuits, corrosion and other problems. According to the national standard GB38031-2020 "Safety Requirements for Power Batteries for Electric Vehicles", the sealing performance of batteries and their components should meet the IP67 standard. Most new energy vehicles have higher sealing performance requirements for batteries and their components, and must meet the IP68 standard, that is, the battery pack can prevent water from entering within the specified water depth and submersion time.
Traditional air tightness testing methods include pressure method and immersion method (water test). The immersion method is to immerse the liquid cooling plate in water and observe whether bubbles are generated to judge the sealing.
Liquid Cooling Plate Water Channel Air Tightness Test Tank
Although the IP68 standard is more stringent, in actual applications, the pressure drop method is often used as the main detection method to meet the IP68 requirements by setting appropriate airtightness detection standards. The pressure drop method determines the airtightness of the battery pack by measuring the pressure change inside the battery pack. When performing airtightness testing, multiple parameters need to be paid attention to, such as inflation pressure, inflation time, pressure stabilization time and leakage rate.
Differential pressure basic principle diagram Direct pressure basic principle diagram
2-Analysis of Liquid Cooling Plate Leakage Problem
With the continuous upgrading of market demand for power battery vehicles, battery energy storage systerms, etc., higher energy density and power density battery packs are widely used. Because of the thermal characteristics of batteries, to ensure the stable operation of core equipment such as batteries and improve energy utilization efficiency, liquid cooling technology is one of the mainstream technical routes for energy storage thermal management, and the air tightness test of the liquid cooling system has become a key link.
Liquid cooling plate leakage is a serious problem: the leakage will hinder the normal flow of the coolant, affect the heat dissipation effect of the liquid cooling plate, and reduce the performance of the equipment; the leakage may also cause aging and damage of system components, reducing the reliability of the system; the leakage may also corrode electronic components and circuits, increasing the risk of equipment failure and fire.
Why does the leakage problem still occur after rigorous air tightness testing during the production and manufacturing process of the liquid cooling plate?
Liquid Cooling System Airtightness Test Process
Liquid seepage may be caused by a variety of factors:
l Tiny cracks and defects. Landscape air tightness testing may detect large leakage channels, but tiny cracks and defects may still exist. These tiny cracks may expand under liquid pressure or high temperature environment, causing liquid seepage.
l Coolant surface tension and wettability differences: When the surface tension of the coolant is low, it is easier to penetrate through tiny gaps. If the surface tension design of the liquid cold plate is unreasonable or the coolant is not properly selected, the liquid seepage problem may be aggravated.
Wettability differences: Different coolants have different wettability on solid surfaces. If the material surface roughness of the liquid cold plate is high or there are microstructural defects, the coolant may penetrate more easily.
l Installation or process problems: If the installation process of the liquid cold plate is not fine enough, or there are defects in the welding, connection and other processes, it may also lead to poor sealing and increase the possibility of liquid seepage.
l Environmental conditions: Changes in temperature, especially in high-pressure environments, may affect the permeability of the coolant. Although these environmental factors may not be considered during air tightness testing, in actual operation, temperature fluctuations may cause seal failure.
l Material aging or fatigue: If the material of the liquid cold plate is used for too long, it may age or fatigue, causing its sealing performance to deteriorate, thereby increasing the risk of liquid leakage.
3-Preventive Measures for Liquid Cooling Plate Leakage
l Improve the design of liquid cooling plate: By optimizing the structure and design of the liquid cooling plate, reduce small cracks and defects, and improve its sealing performance. For example, when welding the module installation beam on the flow channel surface, take anti-leakage measures to avoid coolant leakage.
l Improve the manufacturing process level: In the production process of the liquid cooling plate, high-quality welding processes and materials are used to ensure that the coolant is not easy to penetrate. At the same time, during the assembly process, strictly follow the operating procedures to avoid looseness or incorrect installation.
l Optimize the combination of detection methods to ensure detection efficiency while improving detection accuracy and reducing missed detection rate. The immersion method and pressure drop method are used for air tightness detection, which is simple to operate, economical, and efficient, and is suitable for large-scale routine detection needs. However, the detection accuracy of the two methods is low. The detection accuracy of the pressure drop method is generally a leakage rate of 1×10-4Pa·m³/s, and the accuracy of the detection results is easily interfered by factors such as temperature, humidity, cleanliness, and pressure. Use detection equipment with higher detection accuracy and better effect to increase the detection accuracy to 1×10-6Pa·m³/s, thereby improving the detection effect.
In addition to the preventive measures for the liquid cooling plate itself, it is also necessary to adopt appropriate response strategies in multiple aspects such as coolant selection, seal selection and equipment working environment.
We will regularly update you on technologies and information related to thermal design and lightweighting, sharing them for your reference
Thank you for your attention to Walmate
In heat dissipation design, adopting effective cost-reduction methods can improve the reliability and efficiency of the overall system while reducing unnecessary costs.
1-Derating design reduces costs
Derating design is a design method that intentionally reduces the electrical, thermal and mechanical stresses that components or products are subjected to during operation. In actual production and use scenarios, the stability of electronic equipment can be improved by reducing the stress borne by components.
Schematic diagram of heat dissipation paths for 2D and 3D packaging
l Reduce working stress: During product design and operation, the working stress of components can be reduced by reducing the workload, controlling the operating frequency, limiting the current and voltage, etc.
l Reduce environmental stress: Reduce environmental stress by selecting appropriate component types, layouts, and packaging forms, such as selecting components with a large temperature margin, or using packaging forms with good sealing to reduce the effects of temperature, humidity, and pressure on components.
l Reliability engineering application: reasonable redundant design, fault detection and isolation, etc., further reduce the failure risk of components.
By reducing the stress on components during operation, their power consumption and heat generation can be reduced. When power devices operate under stress conditions lower than their rated stress, their power consumption and heat generation can be reduced, which helps to improve the energy efficiency and reliability of the system. In the long run, derating design effectively increases the life of components, reduces failure rates, reduces maintenance workload, and thus reduces costs.
2-Optimize layout
The working efficiency of the radiator can be significantly improved through reasonable arrangement of thermal components, and a reasonable component layout strategy can achieve a balance between product performance and cost.
l Distribute heat dissipation components: Disperse components that generate large amounts of heat to reduce the heat load per unit area.
l Location that is conducive to heat dissipation: Place the heating element in a location that is conducive to heat dissipation, such as near a vent or the edge of the device.
l Staggered arrangement: During layout, stagger the heating components with other general components, and try to make the heating components principle temperature-sensitive components to reduce their impact on the heat-sensitive components.
l Improve airflow: By changing the direction design and component layout, the airflow path is optimized, the flow rate is increased, and the heat transfer coefficient is improved.
Spacing recommendations between components
3-Choice of cooling method
As the performance of electronic components improves and the degree of integration increases, the power density continues to increase, resulting in a significant increase in the heat generated by the electronic components during operation. When choosing a heat dissipation method for electronic components, the temperature control requirements mainly include the following aspects:
l Temperature range: Different components have different temperature tolerance ranges. For example, high-performance chips such as CPUs have operating temperature requirements between 85-100°C, while some low-power devices can tolerate higher temperatures, so the cooling system must ensure Components operate within a safe temperature range.
l Temperature control accuracy: In some scenarios with strict temperature control requirements, it is necessary to adopt a heat dissipation solution that can accurately control the temperature to avoid component performance degradation or even damage caused by excessively high or low temperatures.
l Ambient temperature: The heat dissipation effect of electronic equipment not only depends on the heat dissipation capacity of the device itself, but is also affected by the surrounding ambient temperature. The heat dissipation design needs to consider changes in ambient temperature and try to keep the device within a suitable temperature range through heat dissipation means.
l Power consumption and reliability: Some low-power electronic components can use natural heat dissipation when they generate low heat. For high-power consumption equipment, it is necessary to wait for the heat dissipation technology of universities to ensure that it maintains normal performance and prolongs operation under high load. service life.
l Sealing and density: In sealed and high-density assembled devices, if the heat generation is not high, you can rely on natural heat dissipation. When components are densely packed and generate large amounts of heat, more effective heat dissipation technologies such as forced heat dissipation or liquid cooling are needed. Liquid cooling and heat pipe technology are used in scenarios with high power consumption and large heat generation, such as high-power electronic components such as traveling wave tubes, magnetrons, and power amplifier tubes, servers and high-power consumption equipment, and three-electric systems of new energy vehicles. Its unique application advantages.
Charging pile air cooling module Charging pile liquid cooling module
When choosing a cooling method for electronic components, it is necessary to comprehensively consider factors such as heat generation and heat flux, ambient temperature and operating temperature, space constraints and thermal isolation requirements, and cost and feasibility. By using appropriate cooling technology and cooling devices to ensure that components operate at a suitable temperature, the cost of system replacement and maintenance can be effectively reduced. In addition, reusing historical projects is also an effective strategy to reduce development and manufacturing costs and improve reliability.
We will regularly update you on technologies and information related to thermal design and lightweighting, sharing them for your reference
Thank you for your attention to Walmate
Energy storage immersion liquid cooling technology is an advanced battery cooling method that uses the efficient thermal conductivity of liquid to achieve rapid, direct and sufficient cooling of the battery, ensuring that the battery operates in a safe and efficient environment. Its basic principle is to completely immerse the energy storage battery in an insulating, non-toxic liquid with heat dissipation capabilities. This technology uses direct contact between the liquid and the battery for heat exchange, thereby quickly absorbing the heat generated by the battery during charging and discharging, and bringing it to the external circulation system for cooling.
Schematic diagram of the principle of a single-phase immersion liquid-cooled energy storage system
As a key component for carrying the battery pack and ensuring that the battery cells work in a suitable environment, the immersion liquid-cooled energy storage Pack box mainly undertakes functions such as carrying the battery pack and coolant, safety protection, and heat conduction. Therefore, in the design of the box structure, it is necessary to comprehensively consider multiple aspects such as airtightness, cooling efficiency, safety, material selection, and processing technology to ensure efficient, safe, and reliable operation of the system. The box structure design is the basis of the entire liquid cooling system.
1-Unified load
The lower box of the immersed liquid-cooled energy storage pack is composed of a bottom plate and side plates. The bottom plate serves as a basic support, and the side plates are fixed around the bottom plate, which together form the main frame of the box. The size of the box should be adjusted taking into account the overall needs and load conditions of the liquid cooling system. In the design of larger-sized boxes, internal partitions or support structures can be reasonably set up to divide the large space into multiple small spaces. force area to improve the uniform load-bearing capacity. In the internal structure, the local load-bearing capacity can be improved by adding support ribs and reinforcing ribs, and a load-sharing structure can also be set up inside the box to balance the load at each corner.
At the same time, in order to reduce the impact of plastic deformation on uniform load-bearing, the processing surfaces of different heights can be designed as the same plane, which can reduce the number of machine tool adjustments and avoid deformation caused by height differences; the width or height of the box can also be increased to disperse the load and reduce deformation.
In addition, the integrated design of the liquid cooling channel and the bottom plate of the box is completed by stir friction welding or laser welding. This design can effectively improve the overall structural strength.
Schematic diagram of the lower box structure of a single-phase immersion liquid-cooled energy storage pack
2-Heat exchange design
Thermal conductivity is an important part of immersion liquid cooling energy storage technology. The design goal is to ensure that the battery can effectively dissipate heat in a high temperature environment, thereby maintaining its performance and safety.
The material of the box should have high thermal conductivity. Commonly used materials are aluminum alloy, copper, and aluminum-based composite materials. The box design also needs to consider the impact of ambient temperature changes. The appropriate thickness of the insulation layer can ensure that the temperature inside the box is within a relatively constant range, thereby improving the overall efficiency of the system.
The structural design of the box directly affects its thermal conductivity. Reasonable flow channel layout ensures that the liquid flows smoothly inside the box and maximizes the contact area, which is the main strategy to improve the thermal conductivity of the box. Multiple flow channels can be set inside the box to increase the coolant circulation path, thereby improving the heat dissipation effect.
Solution 1: single item + plate replacement Solution 2: single item + box replacement
The liquid cooling system includes a cooling medium, a heat-conducting structure, a liquid cooling pipeline and a supporting structure.
In the first scheme, the same or different types of coolants can be selected to be filled into the liquid cooling plate flow channel cavity and the box cavity respectively, and the two cavities are sealed and not connected to each other. In the box cavity, the coolant immerses the battery module, fully contacts it, cools it without flowing, and absorbs the heat on the battery surface by using the good thermal conductivity of the liquid to reduce the temperature rise. In the liquid cooling plate, the coolant is divided into multiple flow channels in the water inlet manifold and enters the cold plate in parallel, and then converges and flows out in the water outlet manifold, which is mainly responsible for taking out the heat and achieving heat dissipation.
In the second scheme, the coolant with low temperature flows in from the bottom or side, and the coolant with high temperature flows out from the top. The coolant circulates in the battery pack, which can effectively and evenly distribute the heat, improve the overall cooling efficiency, and maintain the consistency of the temperature of the battery cell or battery pack.
In order to further improve the cooling effect, a variety of optimization measures can be taken, such as optimizing the liquid flow and circulation method, selecting a coolant with high heat capacity, and improving the temperature distribution of the liquid. These measures reduce heat buildup and energy loss, ensuring the battery operates in an efficiently cooled state.
3-Sealed design
For liquid cooling pack boxes, the fully sealed design is carried out by adopting advanced sealing materials and structures. The sealing design should not only consider air tightness, but also the sealing of liquid media to ensure that the battery cells are leak-free in all directions.
The design should select the appropriate sealing form and shape according to the specific application requirements, and also consider factors such as the leakage freedom, wear resistance, medium and temperature compatibility, and low friction of the seal, and select the appropriate seal type and material according to the detailed specifications.
In addition, the choice of welding process also has a great influence on the sealing performance. For different materials and thicknesses, choosing the appropriate welding method can effectively improve the quality of the weld to ensure the overall strength and sealing of the system.
Finished picture of the lower box of the single-phase immersion liquid-cooled energy storage pack
We will regularly update you on technologies and information related to thermal design and lightweighting, sharing them for your reference.
Thank you for your attention to Walmate.